Transmembrane protein 97 is a potential synaptic amyloid beta receptor in human Alzheimer’s disease
By Martí Colom-Cadena, Jamie Toombs, Elizabeth Simzer, Kristjan Holt, Robert McGeachan, Jane Tulloch, Rosemary J. Jackson, James H. Catterson, Maxwell P. Spires-Jones, Jamie Rose, Lora Waybright, Anthony O. Caggiano, Declan King, Francesco Gobbo, Caitlin Davies, Monique Hooley, Sophie Dunnett, Robert Tempelaar, Soraya Meftah, Makis Tzioras, Mary E. Hamby, Nicholas J. Izzo, Susan M. Catalano, Claire S. Durrant, Colin Smith, Owen Dando, and Tara L. Spires-Jones
Excerpt from the article publishe in Acta Neuropathol 147, 32 (2024). 06 February 2024. DOI: https://doi.org/10.1007/s00401-023-02679-6
Editor’s Highlights
- TMEM97, transmembrane protein 97, is a promising potential synaptic binding partner of amyloid beta (Aβ).
- TMEM97 was recently identified as the gene that codes for the Sigma-2 receptor.
- Sigma-2 receptors have been studied for more than four decades and are drug targets for several conditions including cancer, pain and diverse CNS disorders.
- The ability of Sigma-2 receptor modulators to displace Aβ oligomers from synapses was based off solely pharmacological/functional data, and data indicating that this effect was correlated with PGRCM1 expression.
- Within presynapses and post-synaptic densities, oligomeric Aβ generates a FRET signal with transmembrane protein 97.
- TMEM97 modulator reduces synaptic TMEM97-Aβ FRET signal in a mouse model of Alzheimer’s.
- TMEM97 is a potential Aβ synaptic binding partner in human brain tissue and confirms that Sigma-2 receptor complex allosteric modulator.
Abstract
Synapse loss correlates with cognitive decline in Alzheimer’s disease, and soluble oligomeric amyloid beta (Aβ) is implicated in synaptic dysfunction and loss. An important knowledge gap is the lack of understanding of how Aβ leads to synapse degeneration. In particular, there has been difficulty in determining whether there is a synaptic receptor that binds Aβ and mediates toxicity. While many candidates have been observed in model systems, their relevance to human AD brain remains unknown. This is in part due to methodological limitations preventing visualization of Aβ binding at individual synapses. To overcome this limitation, we combined two high resolution microscopy techniques: array tomography and Förster resonance energy transfer (FRET) to image over 1 million individual synaptic terminals in temporal cortex from AD (n = 11) and control cases (n = 9). Within presynapses and post-synaptic densities, oligomeric Aβ generates a FRET signal with transmembrane protein 97. Further, Aβ generates a FRET signal with cellular prion protein, and post-synaptic density 95 within post synapses. Transmembrane protein 97 is also present in a higher proportion of post synapses in Alzheimer’s brain compared to controls. We inhibited Aβ/transmembrane protein 97 interaction in a mouse model of amyloidopathy by treating with the allosteric modulator CT1812. CT1812 drug concentration correlated negatively with synaptic FRET signal between transmembrane protein 97 and Aβ. In human-induced pluripotent stem cell derived neurons, transmembrane protein 97 is present in synapses and colocalizes with Aβ when neurons are challenged with human Alzheimer’s brain homogenate. Transcriptional changes are induced by Aβ including changes in genes involved in neurodegeneration and neuroinflammation. CT1812 treatment of these neurons caused changes in gene sets involved in synaptic function. These data support a role for transmembrane protein 97 in the synaptic binding of Aβ in human Alzheimer’s disease brain where it may mediate synaptotoxicity.
Introduction
In Alzheimer’s disease, synapse loss is an early event in the aetiology of the disease and is the strongest pathological correlate of cognitive decline [17, 56, 67]. The mechanism(s) underlying synapse degeneration, however, are still largely unknown [10]. We and others have observed that oligomeric amyloid beta (Aβ) peptide causes synaptic dysfunction, accumulates within in synapses, and is associated with synapse loss around plaques [34, 41, 52, 60, 64]. While it is clear that toxicity of tau and changes in non-neuronal cells are also important in disease pathogenesis [24], substantial evidence supports an important role for Aβ in synaptotoxicity early in the disease process in Alzheimer’s [38]. As such, it is important to identify synaptic binding partners of Aβ which may mediate synaptotoxicity in human brain. Disrupting binding of Aβ with synaptic receptors is a promising therapeutic avenue as such interactions are “druggable”, or able to be interrupted with standard pharmacological approaches [14].
Synaptic Aβ binding partners have been identified in cell culture systems and mouse models, but their human relevance is still debated (reviewed in [5, 30, 46, 63]). Among the Aβ binding candidates, cellular prion protein (PrPc) represents the most studied, either alone or through a complex with mGluR5 [37, 62, 72, 79]. Other suggested Aβ binding partners at synapses include the α7-nicotinic receptor [49], Ephrin A4 [73], PSD95 [35, 50, 52] and LilrB2 [32]. An important outstanding question in the field is which of these potential partners binds Aβ in human synapses, as most binding partners have not been validated in Alzheimer’s cases nor using human derived Aβ species [38, 63]. Further, in model systems, Aβ is often overexpressed or applied exogenously, and due to the “sticky” nature of Aβ oligomers, this can result in false positive signals for interacting partners, which has been highlighted as a problem for translation in the field [5].
TMEM97, transmembrane protein 97, is a promising potential synaptic binding partner of Aβ. TMEM97 was recently identified as the gene that codes for the Sigma-2 receptor [3]. Sigma-2 receptors have been studied for more than four decades and are drug targets for several conditions including cancer, pain and diverse CNS disorders [22, 58]; and most notably, a Sigma-2 modulator, CT1812, is in clinical development in Phase 2 trials for Alzheimer’s disease and dementia with Lewy bodies [11, 21]. In the context of Alzheimer’s, in 2014, Izzo and colleagues found that Sigma-2 modulators including CT1812 could displace Aβ synthetic oligomers from their synaptic receptors in cellular models and could improve cognitive deficits in a mouse model of Alzheimer’s [27]. Yi et al.and Mondal et al.similarly found that Sigma-2 receptor ligands prevent neurodegeneration in a worm model expressing human amyloid precursor protein [44, 77].
Little is known about the pathophysiological role of Sigma-2, especially due to its unknown identity until the identification of TMEM97. TMEM97, initially known as MAC30 [47], is overexpressed in some cancers and it is believed to be a key player of cholesterol homeostasis [78] and calcium regulation [8, 74, 77]. Linking this function to Alzheimer’s, in cellular models, TMEM97 has recently been shown to form a ternary complex with Progesterone receptor membrane component 1 (PGRMC1) and LDLR [55] that may control the internalization of monomers and oligomers of Aβ [54]. In addition, our group recently found increased levels of TMEM97 in synaptoneurosomes from Alzheimer’s cases, compared to control, in a proteomics study [25], supporting a potential role in synaptotoxicity in humans.
Until the 2017 discovery that TMEM97 encodes the Sigma-2 receptor [3], the understanding of the ability of Sigma-2 receptor modulators to displace Aβ oligomers from synapses was based off solely pharmacological/functional data, and data indicating that this effect was correlated with PGRCM1 expression. To date, there has been no direct interrogation of whether TMEM97, PGRMC1 and Aβ are found within the same synapses in human brain, whether CT1812 affects TMEM97 binding to Aβ within synapses, or whether PrPC binding to TMEM97 may underlie the ability of Sigma-2 receptor modulators to displace Aβ oligomers. The herein paper addresses these knowledge gaps for the first time.
The study of synapses in the human brain represents a technical challenge due to their small volumes, which are smaller than the diffraction limit of light microscopy, making colocalization studies difficult. In the present work we applied a new approach for the study of the close proximity of proteins in synapses in human post-mortem brain tissue. To visualize the potential interaction between Aβ and potential binding partners at synapses, we combined array tomography [43] and Föster resonance energy transfer (FRET) microscopy [20, 80]. Array tomography allows us to reach a 70nm axial resolution, which enables the identification of single synaptic terminals in three dimensions [31]. The combination of array tomography with FRET enhances the lateral resolution to ~ 10 nm in the selected single synaptic terminals allowing us to determine whether proteins are close enough to be interacting [23, 52].
The current study tests the hypothesis that TMEM97 interacts with Aβ in synapses in human Alzheimer’s brain and that modulating this can recover synaptic phenotypes in model systems. We demonstrate that TMEM97 is a potential Aβ synaptic binding partner in human brain tissue and confirms that Sigma-2 receptor complex allosteric modulator CT1812 can reduce interactions between TMEM97 and Aβ in vivo. These findings shed light on the mechanisms of action by which a Sigma-2 receptor modulator may be acting in the context of Alzheimer’s, and may help further Alzheimer’s therapeutic approaches, in both drug discovery and clinical development. Finally, this study enables further technical advances in the study of the still elusive synaptic structures involved in neurodegeneration.
…
Results
Characteristics of human cases
We used human post-mortem brain samples from inferior temporal gyrus (BA20/21) to investigate proximity of Aβ and synaptic proteins. Details of human cases included are shown in Table 1. Our Alzheimer’s and control cohorts are age (Wilcoxon test W = 29.5, p = 0.13) and sex matched (p = 1 Fisher’s exact test) and matched for APOE4 allele status (Fisher’s exact test p = 0.41). Post-mortem interval (PMI) was slightly longer in Alzheimer’s group (Welch’s t test, t = − 2.36, p = 0.030). Both Braak stage and Thal phase were higher in AD than control group due to our inclusion criteria for the groups (p < 0.01 Fisher’s exact tests).
TMEM97 levels are increased in Alzheimer’s post synapses
We used array tomography to examine synaptic localisation of Aβ, TMEM97 and other potential binding partners. Image stacks were acquired in areas containing amyloid plaques where we previously demonstrated the highest amount of synaptic Aβ. The overall density of TMEM97 positive objects was assessed in the temporal cortex revealing an immunoreactivity pattern of a membrane protein, with widespread presence in grey matter in both Alzheimer’s and control cases (Fig. 1A). The density of TMEM97 objects was significantly higher in Alzheimer’s than control cases (fold increase: 1.56; effect of disease F[1,19.36] = 4.41, p = 0.049, no significant sex differences). This increase was not related to the proximity of an Aβ plaque (Fig. 1B). As previously described [34], post-synaptic terminal density was reduced in AD (Fig. 1B, 7% reduction in PSD density F[1,28.73] = 4.36, p = 0.046, no significant sex differences). Postsynaptic puncta density was further reduced in the vicinity of Aβ plaque cores of Alzheimer’s cases (effect of plaque distance F[4,Inf] = 4.44, p = 0.001, no sex effect, Fig. 1C). As expected, Aβ object density was elevated close to Aβ plaques (effect of plaque distance F[4,Inf] = 47.86, p < 0.0001; Fig. 1D).
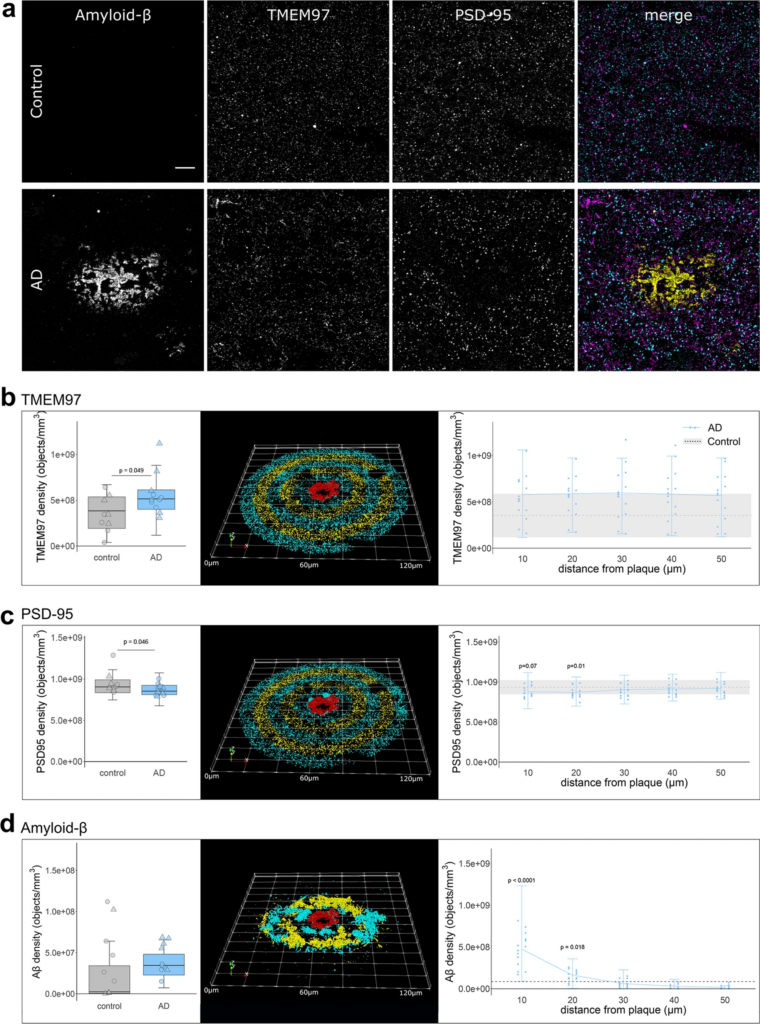
Immunoreactivity pattern and density of TMEM97, Aβ and PSD95. a representative maximum intensity projection images of ten consecutive 70 nm-thick sections from a control and an Alzheimer’s case. Immunoreactivity against Aβ (6E10, yellow), TMEM97 (magenta) and PSD95 (cyan) is shown. Overall density (left) or the density in relation to Aβ plaque cores (right) of TMEM97 (b), PSD95 (c) and Aβ (d) is plotted. The 3D reconstructions were made from 19 consecutive sections of a representative Alzheimer’s case. The Aβ core is shown in red and the objects distributed every 10um bins are coloured. Scale bar represents 10 µm. Boxplots show quartiles and medians calculated from all image stacks in the study. Data points show case means (females, triangles; males, circles). p values on left panels show significant effect of disease (ANOVA after linear mixed effects model). On right panels, p values show Tukey corrected post-hoc significant differences between 50 μm and the indicated plaque distance in the AD data. In D, note the scales are different in the two plots as there is an order of magnitude more Aβ near plaques than when averaged across all images
TMEM97 and Aβ are found in a higher proportion of synapses in Alzheimer’s
We recently described an increase of TMEM97 protein levels in biochemically isolated synaptic fractions from Alzheimer’s brain compared to controls [25], and in multiple previous studies we and others have observed increased levels of in Aβ synapses [29, 34]. In the present study, we confirmed biochemically that both Aβ40 and Aβ42 levels are increased in synaptic fractions of AD brain (Fig. S2). Further, we were able to visualize the synaptic localization of TMEM97 and Aβ using array tomography. The analysis of over 1 million individual synaptic terminals revealed an increased proportion of synapses with TMEM97 in Alzheimer’s when compared to healthy controls (fold increase: 2.10; effect of disease F[1,20.16] = 5.67, p = 0.027, Fig. 2). There were no sex effects in the proportion of synapses containing TMEM97. In line with the hypothesis that TMEM97 is a binding partner of Aβ, we found that Aβ was present in post-synaptic terminals (effect of disease F[1,21.86] = 7.56, p = 0.011, Fig. 2). Interestingly, there was a significant interaction between disease and sex in the proportion of synapses with Aβ (disease* sex interaction F[1,21.86] = 5.1, p = 0.034) with female subjects having more synaptic Aβ. Importantly, that Aβ was found overlapping TMEM97 immunoreactivity in the same post synapses (effect of disease F[1,20.66] = 19.72, p = 0.0002, Fig. 2).
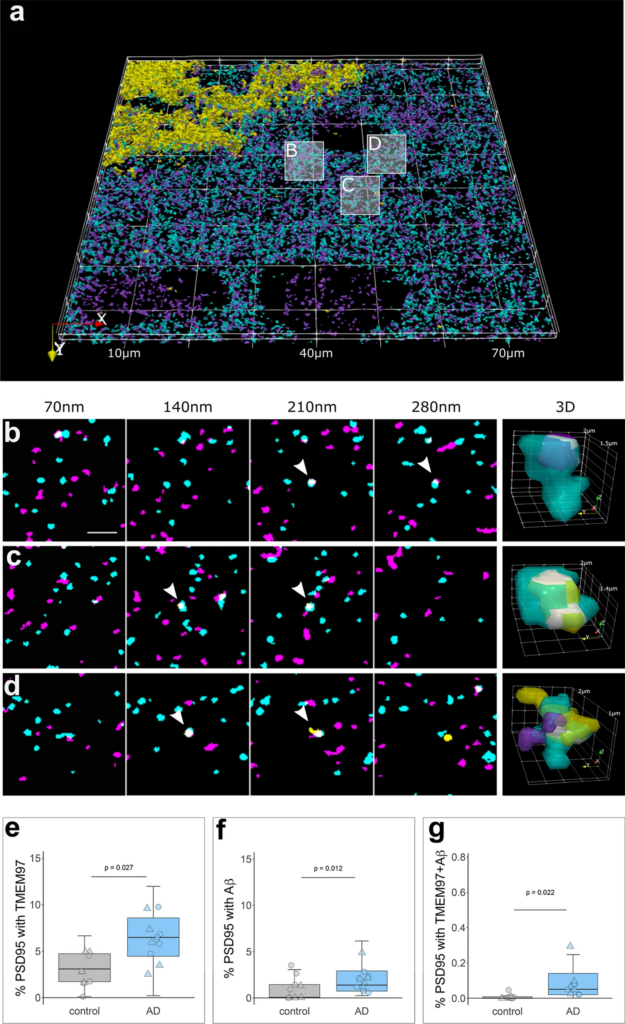
TMEM97 is found at higher levels in Alzheimer’s synaptic terminals compared to healthy controls. 3D reconstructions were made from 20 consecutive 70 nm-thick sections from a representative Alzheimer’s case stained for Aβ (6E10, yellow), TMEM97 (magenta) and PSD95 (cyan). In the top 3D reconstruction (a), three white boxes label the magnified regions that highlight: a PSD95 terminal with TMEM97 (b), a post-synaptic terminal with Aβ (c) and a PSD95 synaptic terminal with both Aβ and TMEM97 (d). In magnified images (b–d), four consecutive sections from the image stack are shown (each 70 nm apart). White arrowheads indicate synaptic localization and a 3D reconstruction (right panel) of the pointed synapse where colocalization is observed (white). Box plots show the percent of post-synaptic terminals that contained TMEM97 (E), Aβ (F), or both (G), in Alzheimer’s and control cases. Boxplots show quartiles and medians calculated from each image stack. Each data points refers to the means of a single human tissue donor (females, triangles; males, circles). p values show ANOVA after linear mixed effects models. Scale bar: 2 µm
Synaptic TMEM97 and Aβ are close enough to generate a FRET signal
After confirming the presence of TMEM97 together with Aβ in synapses of Alzheimer’s cases, and given the ability of Sigma-2 receptors to displace Aβ from neuronal synapses [27], we assessed the proximity of the immunoreactivity of TMEM97 with that of Aβ in synapses of AD cases by FRET. In this single pixel analysis, those areas where the donor (labelling Aβ) and the acceptor (labelling TMEM97) were found overlapping within a PSD95 positive object were quantified in the corrected donor excitation-acceptor-emission image (Fig. S1 and methods for further details). This quantification allowed us to detect FRET only when both donor and acceptor were present in synapses and within approximately 10nm of each other. To determine limitations of the technique, we measured the residual FRET signal in stacks where only the donor or the acceptor was labelled as negative controls, and the maximum FRET signal was determined where the donor and acceptor labelled the same target as the positive control (Fig. 3, green shading on the plot shows the experimental window of detecting FRET signal between negative and positive control levels).
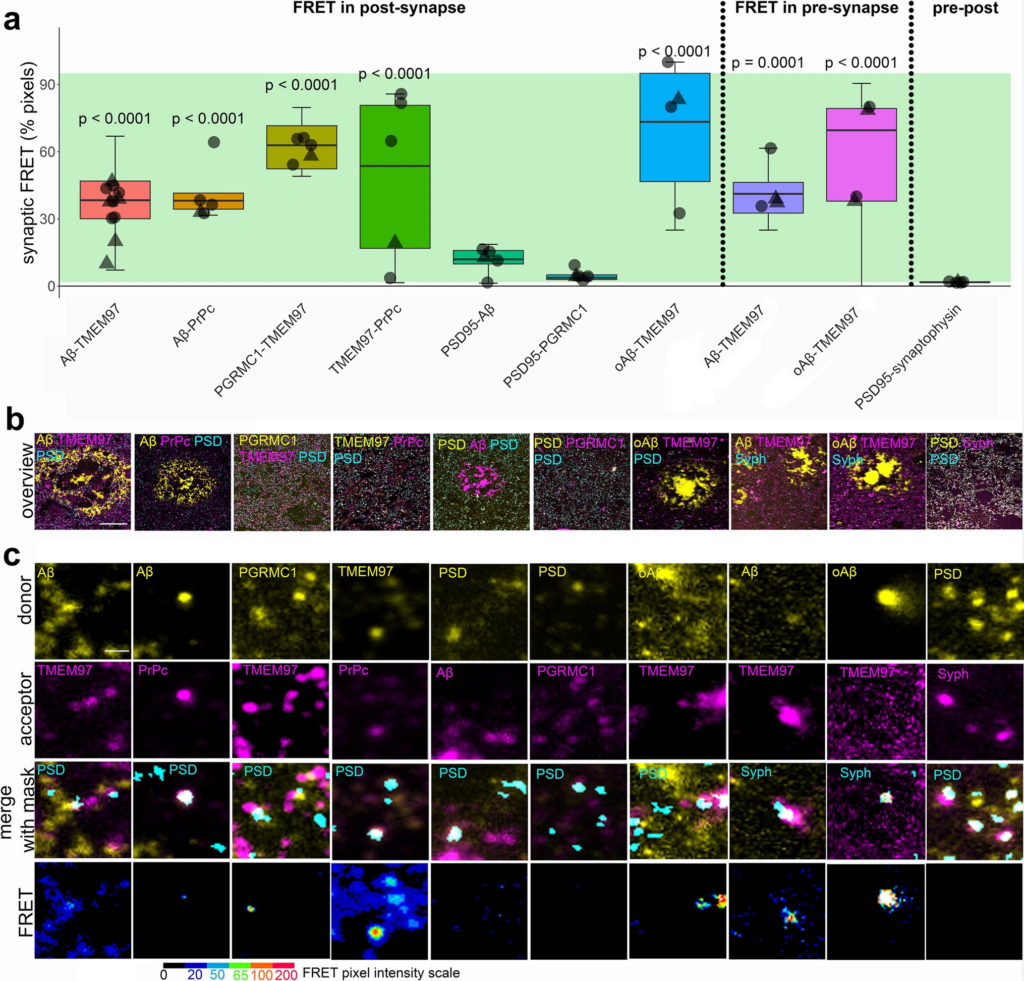
Aβ and TMEM97 are close enough at the Alzheimer’s synapses to generate a FRET effect. Pixels where pairs of interest were colocalized within synaptic puncta were analysed to determine whether they generate a FRET signal. The percent of synaptic pixels where FRET signal was detected by each protein pair are plotted (a). The green bar in the boxplot shows the window of detecting FRET signal defined by the positive control signal where an acceptor fluorophore was applied to the same protein as the donor fluorophore using a tertiary antibody (top) and negative controls where no acceptor fluorophore was present (bottom). Boxplots show quartiles and medians calculated from each image stack. Data points show case means (females, triangles; males, circles). p values show post-hoc Tukey corrected differences between the pair indicated and the biological negative control of PSD-synaptophysin FRET. Images in panel bshow a maximum projection of five consecutive sections showing a 100 × 100 μm overview of the donor channel (yellow) acceptor channel (magenta) and the synaptic channel segmented in three dimensions used as region of interest for FRET (cyan) for each FRET pair used in the study. Panel c shows 5 × 5 μm regions containing examples of donor and acceptor staining in synaptic masks and the generated FRET signal in a single section with intensity represented by colour as in colour scale. Scale bars: 20 µm in b, 1 µm in c
In Alzheimer’s cases, we found that in both post and pre synapses where donor and acceptor were present, Aβ labelled with 6E10 antibody and TMEM97 were close enough to generate a FRET signal (Fig. 3). In post synapses, we also observed FRET between Aβ and PrPc—which has also been observed to bind Aβ in model systems[62]—and between TMEM97 and PGRMC1 which are known to be binding partners in vitro and in human cases [55], and between TMEM97 and PrPc (Fig. 3). Confirming cellular localization of Aβ to synapses, we saw positive FRET signal between Aβ and PSD95 (Fig. 3). This is in support of findings by other groups in which Aβ has been described to interact in some synapses, including in rat neuronal cultures [28] and our previous study using a similar FRET approach in APP/PS1 mice [35, 50, 52]. We confirmed that TMEM97 generates a FRET signal with oligomeric Aβ in both pre and post synapses using NAB61 to label oligomers and fibrillar oligomers of Aβ (Fig. 3).
To confirm that this effect was not occurring in all areas where donor and acceptor are present in the same pixel, we used a biological negative control looking for FRET between PSD95 and synaptophysin which are close but not interacting as they are separated by the synaptic cleft. As expected, there was not a significant FRET signal between these pre and post-synaptic proteins. There was also no FRET signal between PGRMC1 and PSD95 (Fig. 3). In summary, our FRET experiments confirm close proximity within synapses of Aβ and TMEM97, Aβ and PrPc, TMEM97 and PrPc, TMEM97 and PGRMC1 and Aβ and PSD95, but not PSD95 and synaptophysin or PSD95 and PGRMC1, which are robust as both technical and biological negative controls do not show FRET signal.
TMEM97 modulator reduces synaptic TMEM97-Aβ FRET signal in a mouse model of Alzheimer’s
Results from human brain observations suggested that TMEM97 may be a binding partner of Aβ. To determine whether this synaptic binding is reversible in vivo, we used the Sigma-2/TMEM97 receptor complex allosteric modulator CT1812—currently in clinical trials for Alzheimer’s [11, 21]—in a recently described Alzheimer’s mouse model [51]. APP/PS1 + Tau mice (APP-PS1 ± ; MAPT -/-; CKTTA + ; Tg21221) and littermate controls were treated with either vehicle (n = 10 APP/PS1 + Tau, n = 10 control) or CT1812 (10 mg/kg/day given orally, n = 10 APP/PS1 + Tau, n = 10 control), which selectively binds to the Sigma-2 (TMEM97) receptor complex (Fig. 4). The dose delivered to mice was based on our previous study in which the same dose and treatment duration caused recovery in memory in a plaque-bearing mouse model [28].
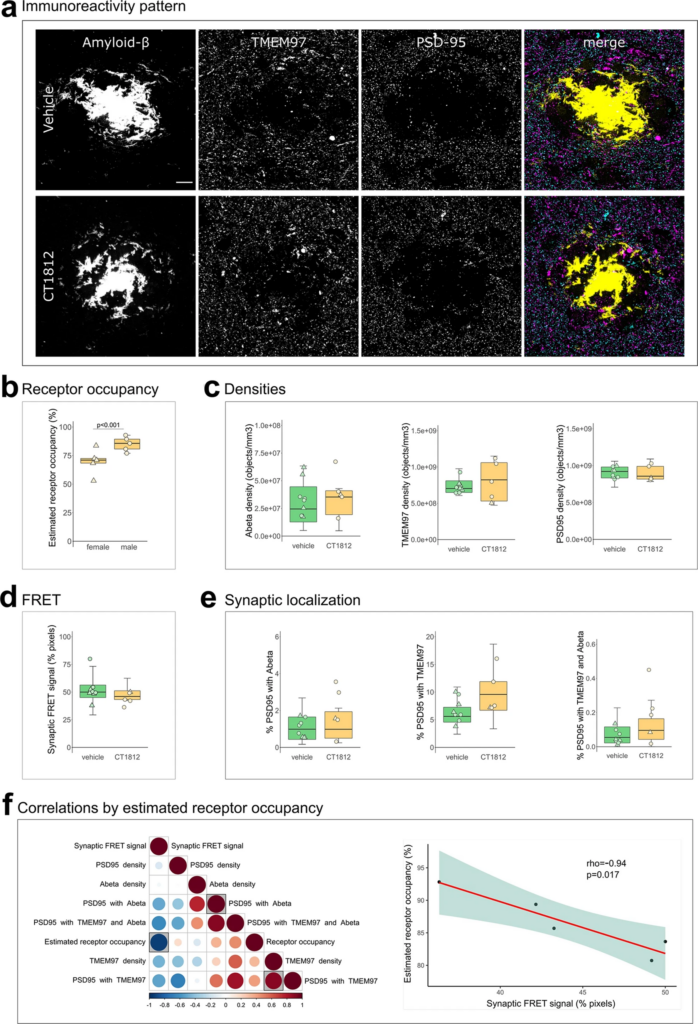
Effect of TMEM97 modulator on synaptic Aβ and TMEM97 in the APP/PS1 + Tau mouse model. Representative images of immunoreactivity patterns found in vehicle or CT1812 treated mice are shown in a. Images show maximum intensity projections of 16 consecutive 70nm-thick sections of cases stained for Aβ (yellow), TMEM97 (magenta) and PSD95 (cyan). b, the estimated percent of receptor occupancy by the drug in the CT1812-treated group. c,quantification of overall densities of the three studied proteins. d, the percent of synaptic pixels that contain both Aβ and TMEM97, and FRET signal. e, the post-synaptic terminals localisation of Aβ, TMEM97, or both. f, Correlations were estimated between measured parameters and a correlation matrix of the assessed variables is shown (left panel) in which the colour and size reflect the rho (scale below the plot) and the statistically significant correlations are highlighted with a shaded square. The correlation between percent estimated receptor occupancy and percent of synaptic FRET signal (right panel) displaying the regression line (red), the 95% confidence interval (green) and the Spearman correlation results (rho, p value). Scale bar: 10µm. Boxplots show quartiles and medians calculated from each image stack. Data points refer to case means (females, triangles; males, circles). Analysis with linear mixed effects models including treatment group and sex interaction
We first estimated the percent receptor occupancy of CT1812 in brain, which was calculated based on the measured brain concentration of the drug (see methods and [27]). We observed a statistically significant sex difference in the percent of estimated receptor occupied by the drug. Male APP/PS1 + Tau mice had an average of 85.13 ± 6.4% estimated receptor occupancy, while females had an average of 69.69 ± 11% (β = 15.44; p < 0.001, Fig. 4B). The increase in drug estimated receptor occupancy in male mice was observed in both genotypes (β = 44.63; p = 0.006, Supplementary Fig S3). This difference could not be explained by any experimental procedures as all animals were given the same dose of compound from the same stock. Body weight, which could affect drug uptake, did not differ between treatment groups (F[1, 36] = 0.74, p = 0.40), but body weight was different between male and female mice with males being heavier (F[1, 36] = 62.33, p < 0.0001). There was no treatment:sex interaction in analysis of body weight nor was there a significant difference in the numbers of male vs female mice in the different treatment groups (Fisher test p = 1, 95% CI = 0.30, 5.04). The treatment of non-transgenic control mice did not affect the density of Aβ, Tau or PSD95 (Supplementary Fig S2A-B), confirming that treatment with this compound was not synaptotoxic. CT1812 treatment in transgenic mice did not change plaque burdens but did reduce astrogliosis in hippocampus (Supplementary Fig S4).
Since it has been reported that drug concentrations above 80% estimated receptor occupancy are effective and concentrations lower than 60% were ineffective [27], the effect on synaptic TMEM97 and Aβ localization was studied on the mice that reached an 80% estimated receptor occupancy threshold (n = 5 APP/PS1 + Tau mice, Table S1). CT1812 did not statistically significantly influence the overall densities of Aβ, TMEM97 or PSD95 nor the synaptic localization of Aβ and/or TMEM97 (Fig. 4A,C,E). When we modelled the effect of treatment and sex on the synaptic FRET signal between Aβ and TMEM97, we did not observe a significant difference between groups (vehicle mean 52.8 ± 12%; treated mean 44.2 ± 5.61%, Fig. 4D). However, the increase of estimated receptor occupancy by the drug significantly correlated with a decrease of synaptic FRET signal between Aβ and TMEM97 (rho = − 0.94, p = 0.017, Fig. 4F).
Taken together, we found that in the CT1812 treated APP/PS1 + Tau mice with estimated receptor occupancy in the therapeutic range, there was a decreased synaptic FRET signal between Aβ and TMEM97, indicating increased distance between the two proteins.
TMEM97 modulator ameliorates Aβ–induced phenotypes in human iPSC neurons
To investigate whether disrupting TMEM97-Aβ interactions protects living human neurons, human iPSC-derived neurons were challenged with the soluble fraction of Alzheimer’s brain homogenate or identical homogenate immunodepleted for Aβ and treated with CT1812 or vehicle to investigate whether disrupting TMEM97-Aβ interactions protects living human neurons. Brain homogenates were added to neuronal media at final concentrations of 90 pM of Aβ in the mock-immunodepleted condition and approximately 8 pM in the immunodepleted condition (detection was at the lower limit of ELISA sensitivity). Immunocytochemistry confirms that TMEM97 is present in homer positive post synaptic puncta in dendritic spines and that when treated with Aβ-containing brain homogenate, Aβ also accumulates in synapses (Fig. 5A). Both cell counts and TUNEL assay showed that the brain homogenate treatments were not cytotoxic compared to media (Fig. 5B, C), which is important as we wish to model synaptic damage, not neuron death, since oligomers of Aβ at physiological concentrations in human brain are thought to cause synaptic damage and not to induce neuron death directly. Cytotoxicity was generally low (< 10%) in all conditions. To confirm that our iPSC-derived neurons were active and look for functional effects of challenging with Aβ containing homogenates and drug treatments, we used calcium imaging after loading neurons with GCaMP6 virus (Supplementary Figure S5). Images of GCaMP6 fluorescence show that neurons were active in all conditions. Quantification of the peak frequency of GCaMP normalized to the baseline condition for each group shows that acute treatment with homogenate or drug does not change between Aβ + or Aβ + homogenate or CT1812 or vehicle. However, both human brain homogenate treated groups are lower than the control (vehicle treatment; Tukey-corrected post-hoc test after linear mixed effects model, p < 0.0001) indicating that exposure to human brain homogenate affects cell function. Pre-incubation with homogenate for 24 h before CT1812 or vehicle treatment induced a significant interaction between Aβ status of the homogenate and drug treatment (F[1,257] = 9.06, p = 0.003) with a significant decrease in peak frequency between Aβ–incubation followed by vehicle treatment and Aβ + followed by CT1812 treatment.
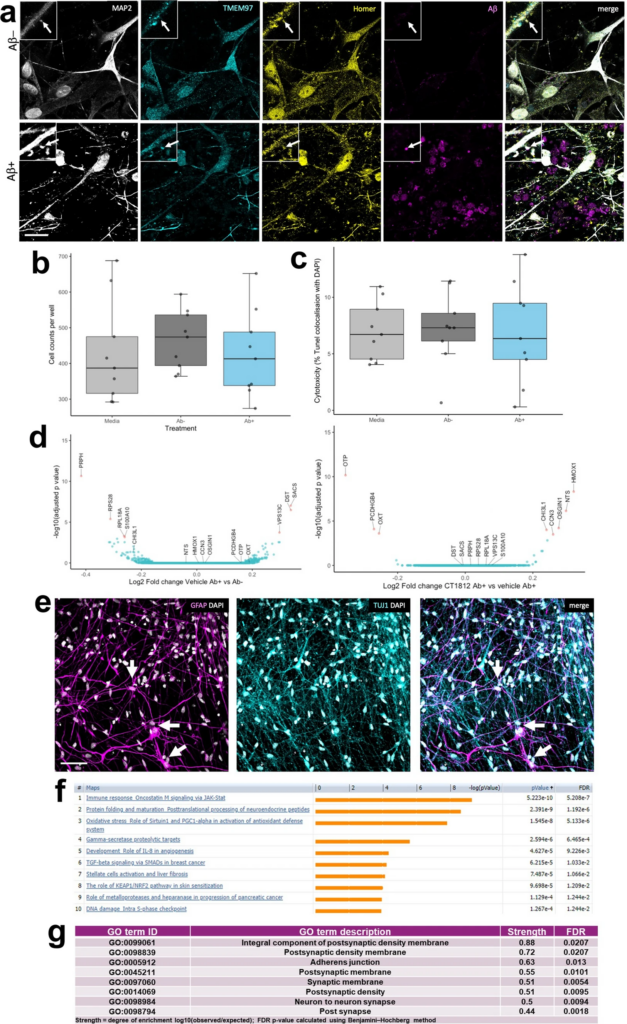
Challenge of human iPSC neurons with Alzheimer’s brain homogenate. (a) Immunocytochemistry for dendrites (MAP2, grey), TMEM97 (cyan), post synapses (homer, yellow), and Aβ (6E10, magenta) reveals that Aβ accumulates in TMEM97-containing post synapses in iPSC-derived human neurons challenged with Alzheimer’s brain homogenate when the homogenate was mock immunodepleted (Ab +), but not when it was immunodepleted for Aβ (Ab−). DAPI positive cell counts (b) and a TUNEL cytotoxicity assay (c) show that brain homogenate treatments do not induce cell death. RNA sequencing reveals seven significantly differentially expressed genes between Ab + and Ab− homogenate treatment (d, differentially expressed genes with adjusted p value < 0.05 shown in pink in volcano plot). When comparing Aβ challenged cultures treated with CT1812 and vehicle, eight genes are differentially expressed (volcano plot in e differentially expressed genes with adjusted p value < 0.05 shown in pink). Staining for astrocytes (GFAP, magenta), neurons (TUJ1, cyan) and nuclei (DAPI, white) reveals that a small proportion of cells in our cultures are astrocytes (arrows) which extend many processes (f). Pathway analysis using MetaCore (unadjusted p value < 0.05) shows enrichment of immune/inflammatory pathways with Aβ treatment compared to immunodepleted Aβ treatment (g). Using STRING protein interaction analysis of Aβ + Drug vs. Aβ + Vehicle conditions (unadjusted p value < 0.05), the top GO Components (sorted by strength) were involved in synaptic biology (top 8 shown, h). Scale bar in panel a 20 μm, insets 10 × 10 μm, Scale bar in panel e 50 μm
RNA sequencing was used to determine gene expression changes after exposing cells to human Alzheimer’s brain homogenate containing soluble Aβ and CT1812 or vehicle. Treatment with Alzheimer’s brain homogenate containing soluble Aβ induced over 4000 differentially expressed genes compared to media treatment. The difference between Aβ− and Aβ + homogenate was much smaller with only 7 differentially expressed genes (adjusted p value < 0.05), 3 upregulated and 4 downregulated (Fig. 5D). Treatment with CT1812 did not significantly change expression of these 7 genes compared to vehicle after Aβ + homogenate. Although not statistically significant, the fold changes of these 7 genes largely changed in the opposite direction with treatment with CT1812 (Table S3). Three of the genes upregulated by Aβ + homogenate challenge are expressed in neurons and implicated in neurodegenerative diseases (DYS—dystonin, SACS—sacsin, and VPS13C—vacuolar protein sorting 13). Eight genes were differentially expressed with CT1812 treatment after Aβ + homogenate challenge (Fig. 5E, Supplementary Table 3). Several of the transcripts regulated by CT1812 are expressed in astrocytes, which are observed in small numbers in our cultures (averaging 16% GFAP positive cells in the 200 day old cultures used, Fig. 5F). One of the transcripts significantly changed by CT1812 treatment, CHI3L1, which encodes YKL-40 protein, is expressed in astrocytes and is an interesting biomarker of inflammation in Alzheimer’s disease [48].
Although only a handful of transcripts reached a statistically significant change after correcting for multiple testing, pathway analysis using a less strict criteria of p < 0.05 unajdusted p values to look for patterns of expression changes using MetaCore, revealed enrichment of immune/inflammatory pathways with Aβ treatment compared to immunodepleted Aβ treatment. These included the JAK/STAT pathway known to be involved in astrocyte differentiation and function, indicated by Leukemia Inhibitory Factor (LIF) and Leukemia Inhibitory Factor Receptor (LIFR) enrichment, a known pro-inflammatory cytokine involved in the differentiation of neuronal precursor cells into astrocytes (Fig. 5F). STRING (Version 11.5) protein interaction analysis of Aβ + Drug vs. Aβ + Vehicle conditions (unadjusted p value < 0.05) confirmed the findings of TMEM97 localization to synapses. The top 8 GO Components (sorted by strength) were involved in synaptic biology (Fig. 5G). Further, “Synapse” was the top UniProt Keyword of the Aβ + Drug vs. Aβ + Vehicle (unadjusted p < 0.05), with a strength of 0.54 and a FDR of 0.00019. Further, biological pathways changed by CT1812 vs vehicle treatment after challenge with Aβ homogenate include several processes important for synaptic function (Supplementary Table 4). Overall, differential expression data and functional imaging support a localization of Sigma-2 receptor at the synapse, and suggest a role for CT1812 in modulating inflammatory pathways, perhaps indirectly by glia that may detect changes in synapse health or activity.
Discussion
In the present study, we visualized TMEM97 within individual synapses in human brain. In Alzheimer’s brain, TMEM97 levels were increased in synapses, and TMEM97 was found in close enough proximity to Aβ to be binding. Further we confirm in mouse and human iPSC models that Aβ is in close proximity to TMEM97 in synapses and that CT1812 treatment to disrupt this interaction is beneficial.
TMEM97 (Sigma-2) has been previously linked to Alzheimer’s, through in vitro and in vivo pharmacological modulation studies and with regard to a change in expression levels on synapses in Alzheimer’s patients. More specifically, in cellular models, treatment with modulators or knocking out Sigma-2 receptor (S2R) constituents TMEM97 or the co-receptor PGRMC1 reduces the internalization of Aβ [27, 54]. In an animal model with Alzheimer’s-like plaque deposition, TMEM97 (Sigma-2) modulators improved cognitive deficits [27, 77]. In human cases, we observed TMEM97 is increased in biochemically isolated synapses of Alzheimer’s patients compared to age-matched controls, using an unbiased proteomic approach [25]. Those findings and the fact that TMEM97 modulators are pharmacologically well studied, have brought the use of TMEM97 modulators into Phase II clinical trials for Alzheimer’s treatment [11, 21].
However, the relationship between Aβ and TMEM97 in human synapses was not previously clear. Our current results support a mechanistic explanation that includes a potentially direct interaction between Aβ and TMEM97, as suggested by the FRET findings (Fig. 6). Importantly, we found that this potential interaction may occur at the synapses, believed to be the earliest affected structure in the context of Alzheimer’s and the best pathological correlate of the characteristic cognitive decline [17, 56, 67]. Taken together, these findings link a therapeutic target (TMEM97) with a suspected pathological peptide (Aβ) in a key cellular structure (the synapse).
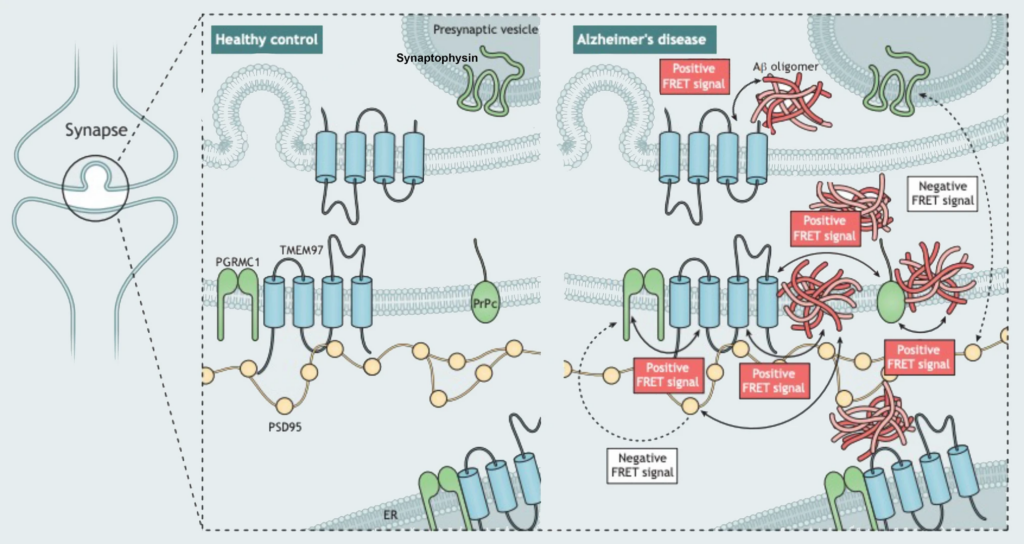
Model of post-synaptic interactions of Aβ. Based on our study, we observe that Aβ is in close proximity to TMEM97, PSD95, and PrPc. Also, TMEM97-PGRMC1 and TMEM97-PrPc were found close enough to generate FRET signals. There was no FRET signal generated between PGRMC1 and PSD95 nor between PSD95 and synaptophysin which should not be in close enough proximity to generate a signal. These data are consistent with Aβ being a binding partner of these synaptic proteins either at the synaptic membrane or potentially within the post-synapse at spine apparatus
The relationship between Aβ and synapses has been widely studied [19, 71]. It has been shown that Aβ can be found in synapses of Alzheimer’s cases [6, 29, 34, 51, 65, 66], but the mechanisms by which Aβ induces synaptic toxicity remain unclear. The study of synaptic binding partners of Aβ yielded many candidates—reviewed in [30, 46, 63]. The most studied binding partner is the PrPc, which through a cascade involving a complex with mGluR5 may lead to toxicity independently or via tau [37, 62, 72, 79]. Other binding partners that have been suggested to bind Aβ include the α7-nicotinic receptor [49], Ephrin A4 (EphA4) [73], PSD95 [35, 50, 52] or LilrB2 [32]. It is likely that Aβ is in fact interacting with more than one protein [62]. Due its hydrophobic nature, Aβ binds to lipid membranes interrupting membrane fluidity and destabilizing several membrane receptors [38]. It is relevant to note that most binding partners have not been studied in human brain nor using human derived Aβ species [38, 63]. Further, while Aβ fibrils bind non-specifically to a variety of surfaces, and Aβ monomers bind to several receptors when applied exogenously [5], Aβ oligomers have been shown to bind saturably to a single site[27, 62] suggesting specific pharmacological interactions with receptors. Therefore, it has been tricky to determine which Aβ binding partners are relevant in living human brain. The structural state of Aβ labelled with 6E10 antibody(monomer, oligomer or fibril) recognised in the present array tomography studies is not clear, which is a limitation of the study, but we have confirmed the interaction of TMEM7 with NAB61, an antibody that preferentially recognises fibrillar oligomers and reacts with synaptic oligomeric Aβ [34]. Within the limitations of the technique, we are able to observe proximity of synaptic proteins to a degree that has not previously been possible within human synapses. There will be synaptic protein in cell bodies of neurons where they are produced before transport to synapses; however, with our technique, we do not observe somatic labelling of PSD95, rather we observe discrete puncta that are opposed to presynaptic terminal labelling [12].
Here we observe that in human synapses, TMEM97 and Aβ are close enough to generate a FRET effect, an observation that allows us to define close proximity, but does not conclusively show a direct interaction. The fact that we also found a FRET effect between Aβ and PrPc in the same cases is in line with previous observations [37, 62], and reinforces the idea of multiple synaptic binding partners of Aβ at the synapses of Alzheimer’s cases. This is important for the field as both modulating TMEM97 with CT1812 and modulating PrPc rescue cognition in plaque-bearing transgenic mice [15, 27], and these data indicate their beneficial effects could be through a similar mechanism. Given that TMEM97 and PrPc and PrPc and Aβ interact, and given that PrPc has been well characterized to bind Aβ, while it is possible that the interaction with TMEM97 and Aβ is direct, it is also possible that the FRET signal between TMEM97 and Aβ is through a direct interaction of TMEM97 with PrPc, thereby enabling a FRET signal with TMEM97 and Aβ, which is bound to PrPc. With the current methodology, we cannot conclusively determine the proportion of Aβ bound to each potential receptor in synapses but our data do support the idea of protein complexes interacting with Aβ. It is possible that some of the binding partners we observe are acting intracellularly with Aβ after internalization into post synapses.
How this interaction may be leading to synaptic dysfunction and subsequent neurodegeneration is less clear. Several mechanisms have been proposed linking Aβ and synaptic dysfunction involving excitatory imbalance [10, 38]. The fact that we found increased levels of TMEM97 in human Alzheimer’s cases and a close proximity between TMEM97 and Aβ at the synapses led us hypothesise that TMEM97 may be involved in the pathogenesis of Alzheimer’s and synaptic dysfunction. In the Alzheimer’s mouse model included in this study we were able to see a reduction of the Aβ potential binding to TMEM97—as reflected by the decrease of FRET signal—associated with increased estimated receptor occupancy of the investigational drug CT1812, only when using the 80% receptor occupancy threshold for which has been previously reported to be required for efficacy.
Given that variable levels of CT1812, both under and over the 80% RO threshold, were observed in this study, it is not surprising that there was no statistically significant change in mean densities of Aβ, TMEM97 or PSD95 or the synaptic localization of Aβ and/or TMEM97 in the CT1812 treated group compared to vehicle. Post-hoc analyses however focusing on changes that might be mediated in animals for which > 80% RO was achieved was in support of efficacy studies which found this threshold must be achieved for efficacy to be observed: that the increase of estimated receptor occupancy by the drug significantly correlated with a decrease of synaptic FRET signal between Aβ and TMEM97 (rho = − 0.94, p = 0.017, Fig. 4F).
The inverse correlation of increasing CT1812 levels with decreased interaction between Aβ and TMEM97 is consistent with the mechanism of action ascribed preclinically, showing a reduction in oligomeric Aβ binding to neuronal synapses in the presence of Sigma-2 receptor modulators [27], and is supportive that CT1812 dosed orally can engage the target, the TMEM97 (Sigma-2) receptor, in the brain, in a drug exposure-dependent manner.
Notably, only five treated mice exhibited drug concentrations above the 80% estimated receptor occupancy, the drug concentration threshold previously defined as effective [27]. The low number of mice with high levels of drug make us cautious about the correlation found while highlight an unexpected finding: a statistically significant increase in drug concentration in male when compared with female mice. None of the variables controlled in the present study could explain the drug concentration differences between males and females and therefore we hypothesise that sex-related biological differences in mouse may be underlying the drug metabolism or blood brain barrier penetration. This is the first study in which CT1812 has been administered to animal models in food, however human clinical trials suggest no difference in CT1812 pharmacokinetics in a fed or fasted state [21]. It is important to note that Izzo and colleagues found an improvement of cognition in mice exhibiting more than 80% estimated receptor occupancy, something only seen in one female of our study [27]. Further, Izzo and colleagues included only male mice in the study, and therefore the present findings on female mice should be taken into consideration to ensure the efficacy of treatments in future studies. These findings may be in line with the increasing body of literature describing sex differences in mice models of Alzheimer’s that may or may not be translated to human cases [1, 2, 16].
Although we could see a reduction in the synaptic FRET signal of Aβ with TMEM97 in the > 80% estimated receptor occupancy group, and a significant correlation with drug brain concentration in the treated group, the treatment of the Alzheimer’s mouse model with TMEM97 modulators did not result in a recovery of synaptic densities nor a decrease of Aβ synaptic localization. CT1812 has been previously demonstrated to selectively displace Aβ oligomers, but not monomer, from synaptic receptor sites and facilitate its clearance out of the brain [27], suggesting that the Aβ that is interacting with TMEM97 observed in this study may be predominantly fibril as opposed to oligomeric. However, disrupting this interaction between TMEM97 and Aβ may be sufficient to improve synaptic function, without requiring a detectable change in synapse density, which could explain the behavioural recovery seen in previous mouse studies with CT1812 treatment [27]. Alternatively, the 28 day treatment period used here may not have been sufficiently long to observe a change in synaptic density; previous studies demonstrating CT1812-mediated improvement in cognitive performance were conducted following 9–10 weeks of administration [27]. Lastly, whereas data herein were generated in the APP/PS1 + Tau mouse model, behavioural data was generated in the Swedish London APP model and it’s possible the forms of Aβ most abundant or relative ratios of oligomeric and fibril forms of Aβ are different across Alzheimer’s models [27].
A previously published model of CT1812’s mechanism of action proposes that the Sigma-2 receptor complex regulates other Aβ oligomer receptors (composed of LilRB2, NGR and PrPc), and when CT1812 binds to TMEM97, allosteric interactions between the Sigma-2 receptor and the oligomer receptor change the oligomer receptors’ shape, destabilize the binding pocket, and increase the off-rate of Aβ oligomers from their receptor. Therefore suggesting that CT1812 does not compete directly with oligomers at the same site [28]. In tumor cells, the canonical Sigma-2 ligand DTG binds to Sigma-2 receptors at a location on the TMEM97 protein [3], and CT1812 displaces radiolabeled DTG binding, but the precise binding location of CT1812 has not been directly determined. Our data indicate that TMEM97 and Aβ are in close proximity where they could be binding, but we cannot rule out that Aβ may be binding to other nearby proteins instead of directly interacting with TMEM97.
In conjunction with FRET showing a synaptic localization, transcriptomic and functional data from human iPSC-derived neuronal cultures treated with soluble fraction of Alzheimer’s brain homogenate with the Sigma-2 modulator CT1812 support a synaptic localization and mechanism. UniProt pathway analysis identified “Synapse” as the most significant term in transcripts altered by CT1812 and the top gene ontology terms important are synaptic and include post-synaptic density and post-synaptic membrane. Of the eight transcripts regulated by CT1812 using a highly stringent statistically criterion (B-H adjusted p < 0.05) was Protocadherin gamma-B4 (PCDHGB4), a cell adhesion molecule on neuronal synapses, which is involved in synaptic maturation and stabilization [36]. Other amongst the eight top-most significant transcripts altered suggest a role for CT1812 in modulating glia. In the cultures studied, ~ 16% of cells were GFAP + astrocytes and data therefore represents a context of cortical neuron and astrocyte interaction. One of the transcripts significantly changed by CT1812 treatment, CHI3L1, which encodes YKL-40 protein, is expressed in astrocytes and is a biomarker of inflammation in Alzheimer’s disease [48]. Beyond the transcript level view, pathway analysis using MetaCore (unadjusted p value < 0.05) revealed enrichment of astrocytic and immune/inflammatory pathways with Aβ treatment compared to immunodepleted Aβ treatment, including the JAK/STAT pathway known to be involved in astrocyte differentiation and function, indicated by LIF and LIFR enrichment, a known pro-inflammatory cytokine involved in the differentiation of neuronal precursor cells into astrocytes (Fig. 5F). We also see in mice that treatment with CT1812 reduces astrogliosis in hippocampus, further supporting an important role for astrocytes in protecting synapses and ultimately cognition. Together, data suggest a role for CT1812 in modulating inflammatory pathways, perhaps indirectly by glia that may detect changes in synapse health or activity. It is possible that some of the TMEM97- Aβ interaction we observed in post synapses contributed to synapse degeneration by glial engulfment as we have recently observed in both human post-mortem tissue and human cellular model systems [70]. In line with the hypothesis of Aβ binding to lipid membranes [38], TMEM97 is thought to be an endo-lysosome-related protein which has itself been linked to cellular toxicity and is essential for the internalization of cholesterol molecules like LDL through the formation of a complex with PGRMC1 [4, 55]. Our transcriptomic data from human iPSC-derived neurons challenged with Alzheimer’s brain derived Aβ confirms neuroinflammatory and neurodegenerative related changes with Aβ challenge. Interestingly, in our plaque-bearing mice, CT1812 treatment reduced astrocyte burden in hippocampus, indicating a potential effect of the drug on glial responses.
CT1812 treatment of human iPSC-derived neurons caused multiple changes in transcripts involved in synaptic function, consistent with the ability of CT1812 to influence synapses and potentially ameliorate damage in Alzheimer’s. Our functional data using calcium imaging similarly showed an interaction between CT1812 and Aβ treatment, however, these results should be interpreted with caution as the cells after 24 h of pre-incubation with human brain homogenate did not appear as healthy as the cells which had acute treatment, and we only had capacity to image 1–2 cell lines per group (albeit with hundreds of imaged objects) in the 24 h condition. However, these data do conclusively demonstrate that our iPSC-derived neurons are active at 180 days post-differentiation—the time point when RNA was also collected for sequencing—and that acutely across lines from 3 donors that CT1812 treatment does not cause excessive excitation.
In summary, in human Alzheimer’s brains we found increased synaptic levels of TMEM97 and close colocalization of TMEM97 with Aβ in synapses. This supports the idea that TMEM97 is a synaptic binding partner for Aβ either directly or indirectly, which is important as this interaction can be modulated by drugs.