Highly specific σ2R/TMEM97 ligand FEM-1689 alleviates neuropathic pain and inhibits the integrated stress response
By Muhammad Saad Yousuf, James J. Sahn, Hongfen Yang, Eric T. David, Stephanie Shiers, Marisol Mancilla Moreno, Jonathan Iketem, Danielle M. Royer, Chelsea D. Garcia, Jennifer Zhang, Veronica M. Hong, Subhaan M. Mian, Ayesha Ahmad, Benedict J. Kolber, Daniel J. Liebl, Stephen F. Martin, and Theodore J. Price
Excerpt from the article published in Proceedings of the National Academy of Sciences, 2023/12/20, 120 (52) e2306090120, DOI: 10.1073/pnas.2306090120
Editor’s Highlights
- Neuropathic pain, which is caused by an injury or disease of the somatosensory nervous system, affects approximately 10% of the population.
- The sigma 2 receptor (σ2R) was recently identified in 2017 as transmembrane protein 97 (TMEM97).
- TMEM97 mRNA is expressed in the human and mouse dorsal root ganglia.
- The signaling effects of σ2R/TMEM97-binding compounds may depend on the pathway that is measured, for instance, LDL uptake was reduced to TMEM97KO levels in HeLa cell regardless of whether cells were treated with σ2R/TMEM97 “agonists” or “antagonists”.
- The analgesic effects of a σ2R/TMEM97 ligand in mice of both sexes require direct modulation of σ2R/TMEM97, not σ1R or any other protein or receptor.
- Modulation of σ2R/TMEM97 leads to the inhibition of integrated stress response (ISR) in mouse and human neurons.
- FEM-1689 inhibits the ISR and promotes neurite outgrowth in a σ2R/TMEM97-dependent fashion.
Significance
Neuropathic pain is a major medical problem that is poorly treated with existing therapeutics. Our findings demonstrate that targeting σ2R/TMEM97 with a modulator reduces pain hypersensitivity in a mouse model with exquisite selectivity. We also identify integrated stress response (ISR) inhibition as a potential mechanism of action that links the receptor to cellular signaling events that have preclinical and clinical validation for pain relief. Our work suggests that σ2R/TMEM97 can be selectively engaged by specific small molecules to produce ISR inhibition in a subset of cells that are critical for neuropathic pain. σ2R/TMEM97-targeted therapeutics thus have the potential to offer effective pain relief without engagement of opioid receptors.
Abstract
The sigma 2 receptor (σ2R) was described pharmacologically more than three decades ago, but its molecular identity remained obscure until recently when it was identified as transmembrane protein 97 (TMEM97). We and others have shown that σ2R/TMEM97 ligands alleviate mechanical hypersensitivity in mouse neuropathic pain models with a time course wherein maximal antinociceptive effect is approximately 24 h following dosing. We sought to understand this unique antineuropathic pain effect by addressing two key questions: do these σ2R/TMEM97 compounds act selectively via the receptor, and what is their downstream mechanism on nociceptive neurons? Using male and female conventional knockout mice for Tmem97, we find that a σ2R/TMEM97 binding compound, FEM-1689, requires the presence of the gene to produce antinociception in the spared nerve injury model in mice. Using primary mouse dorsal root ganglion neurons, we demonstrate that FEM-1689 inhibits the integrated stress response (ISR) and promotes neurite outgrowth via a σ2R/TMEM97-specific action. We extend the clinical translational value of these findings by showing that FEM-1689 reduces ISR and p-eIF2α levels in human sensory neurons and that it alleviates the pathogenic engagement of ISR by methylglyoxal. We also demonstrate that σ2R/TMEM97 is expressed in human nociceptors and satellite glial cells. These results validate σ2R/TMEM97 as a promising target for further development for the treatment of neuropathic pain.
Introduction
Neuropathic pain, which is caused by an injury or disease of the somatosensory nervous system, affects approximately 10% of the population and is the leading cause of high-impact chronic pain (1). Management of neuropathic pain is a major clinical challenge because available drugs not only have limited efficacy, but they also elicit serious side effects. There is a significant need for novel drugs that alleviate neuropathic pain through nonopioid and nonaddicting mechanisms and have improved side effect profiles.
The sigma 2 receptor (σ2R) was identified in 2017 as transmembrane protein 97 (TMEM97) (2). We found that several small molecules that bind selectively to σ2R/TMEM97 produce strong and long-lasting antineuropathic pain effects from spared nerve injury (SNI) in mice (3), a finding that was independently replicated with structurally distinct molecules (4). Although the biological function of σ2R/TMEM97 is not well understood, it is a transmembrane protein that is associated with the endoplasmic reticulum (ER) and plays a role in calcium signaling (5, 6) and cholesterol trafficking and homeostasis (7–11). 20(S)-Hydroxycholesterol was recently identified as an endogenous ligand for σ2R/TMEM97 (12). The role of σ2R/TMEM97 in disease pathology has historically been focused on cancer (13), but it is also implicated in neurodegenerative diseases including Alzheimer’s disease (14–17) and Parkinson’s disease (18). Pharmacological targeting of σ2R/TMEM97 has neuroprotective effects in a number of models of neurodegenerative conditions, including traumatic brain injury (19), Huntington’s disease (20), and retinal ganglion cell degeneration (21).
The mechanism by which modulation of σ2R/TMEM97 alleviates neuropathic pain is not known. Given the localization of σ2R/TMEM97 at the ER, a primary hypothesis tested in our work is whether σ2R/TMEM97 targeting may reduce pain via interference with the integrated stress response (ISR), which includes ER stress. The ISR is an adaptive response to cellular stressors such as accumulation of misfolded proteins, lipid and oxidative stress, amino acid and heme deprivation, and viral infection (22, 23). A canonical signaling event associated with the ISR is phosphorylation of eukaryotic initiation factor 2α (eIF2α) in response to cellular stress conveyed by four kinases: protein kinase R (PKR), PKR-like ER kinase (10), heme-regulated inhibitor (HRI), and general control nonderepressible 2 (GCN2). Phosphorylation of eIF2α inhibits global protein synthesis and promotes the translation of mRNAs such as activated transcription factor 4 (ATF4). We and others have demonstrated that the induction of the ISR is associated with neuropathic pain caused by traumatic nerve injury (24, 25), metabolic disorders (26–28), and autoimmune disorders (29–31).
Another key question is whether the antineuropathic pain effects of σ2R/TMEM97 ligands are specifically due to their binding to σ2R/TMEM97 because such compounds can also have substantial activity at the sigma 1 receptor (σ1R), a receptor that also promotes antinociception in animal models (32–35). We used a knockout (KO) mouse of the Tmem97 gene, and a small molecule, FEM-1689, that has improved selectivity for σ2R/TMEM97 to test the hypothesis that σ2R/TMEM97 is causatively linked to antinociception in mouse neuropathic pain models. Indeed, the antinociceptive effect of FEM-1689 in the SNI model is completely absent in TMEM97KO mice. Our findings also show that FEM-1689 inhibits the ISR in a σ2R/TMEM97-dependent fashion in mouse and human DRG neurons. This work provides a strong mechanistic case for targeting of σ2R/TMEM97 as the basis of an approach to treat neuropathic pain.
Results
TMEM97 mRNA Is Expressed in the Human and Mouse Dorsal Root Ganglia.
To assess whether σ2R/TMEM97 is expressed in human nociceptors, we performed RNAscope in situ hybridization using human dorsal root ganglia (DRG) obtained from organ donors. We found that TMEM97 is expressed in all classes of human sensory neurons including SCN10A(Nav1.8)-positive, putative nociceptors (Fig. 1 A–C). Approximately 70% of all human DRG neurons expressed SCN10A transcripts, consistent with our previous observations (36), and all neurons evaluated expressed TMEM97 mRNA (Fig. 1C). Cell size distribution matrix of TMEM97expressing neurons (average = 74 µm) showed that TMEM97 mRNA was not restricted to a subpopulation of neurons (Fig. 1D). Upon further investigation, we found that FABP7-positive satellite glial cells also express TMEM97 (Fig. 1E). We identified and quantified TMEM97-expressing neuronal subpopulations in the human DRG using our previously published human DRG spatial RNA sequencing dataset (Fig. 1F) (36). We validated our findings that TMEM97 is expressed across all neuronal subtypes in the human DRG with particularly high expression in proenkephalin (PENK)-positive nociceptors and Aδ LTMRs (Fig. 1F). Mouse DRG neurons and satellite glial cells express Tmem97 mRNA (SI Appendix, Fig. S1A) with a nearly identical pattern to what is seen in the human DRG. Moreover, previously published single-cell RNA sequencing data from mice shows that Tmem97 is expressed in all DRG neuronal subtypes with particularly high expression in nonpeptidergic neurons, Schwann cells, and satellite glial cells (37). The recently developed harmonized dataset of rodent, nonhuman primate, and human DRGs show that TMEM97 is expressed in all sensory neuron types (SI Appendix, Fig. S1B) (38).
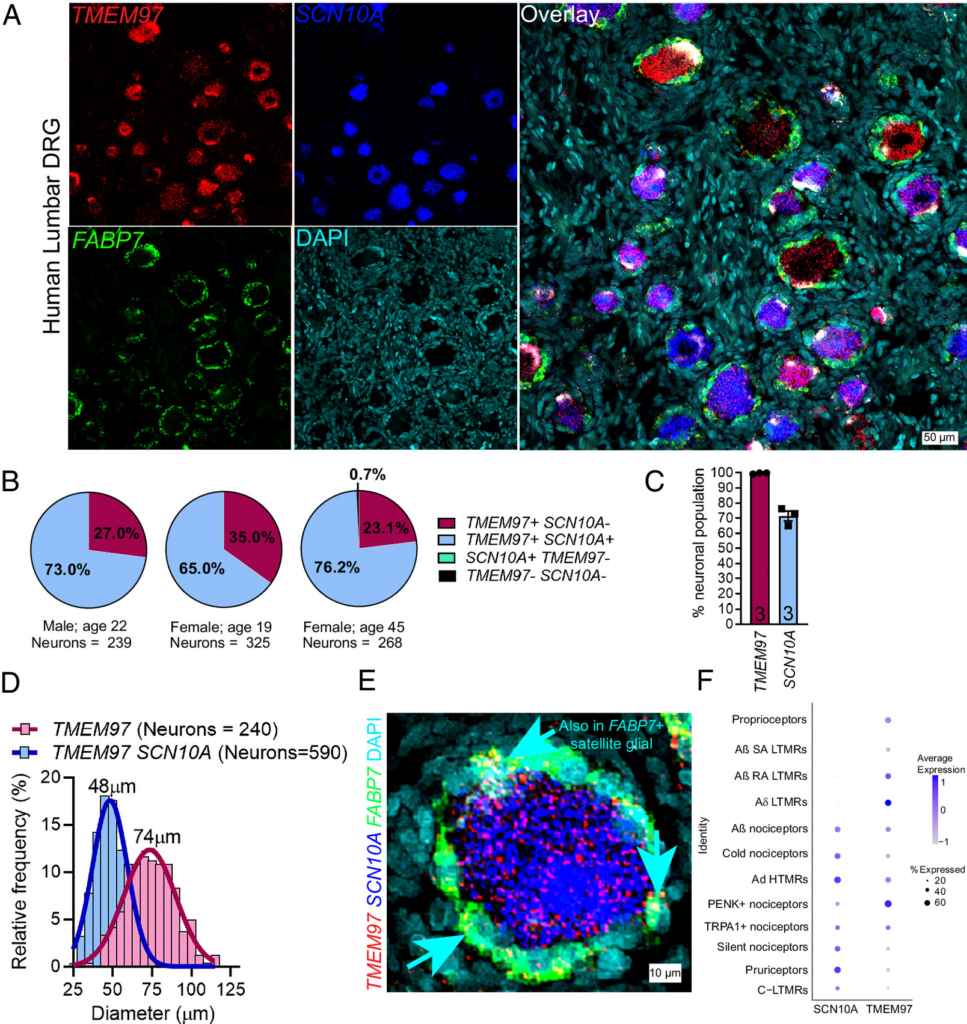
TMEM97 gene is expressed in human DRG. (A) RNAScope in situ hybridization experiments using lumbar DRGs obtained from organ donors. (B and C) Across three donors (one male and two females), we found that nearly all DRG neurons (>99%) expressed TMEM97, and notably, all SCN10A-positive nociceptors expressed TMEM97. (D) TMEM97-positive neurons were distributed across all cell sizes. (E) Upon further investigation, we also identified TMEM97 transcripts in FABP7-positive satellite glial cells. (F) Our previously published (36) analysis of near single-cell RNA sequencing of human DRGs showed that TMEM97 is expressed across all neuronal cell types in the ganglia including nociceptors, low-threshold mechanoreceptors (LTMRs), and proprioceptors. TMEM97 transcripts were notably enriched in proenkephalin (PENK)+ nociceptors and Aδ LTMRs.
Identification of FEM-1689 as a Potent σ2R/TMEM97 Binding Ligand.
Methanobenzazocines and norbenzomorphans represent two distinct chemotypes of biologically active ligands, including UKH-1114, JVW-1034, SAS-0132, and DKR-1677, that bind selectively to σ2R/TMEM97 (SI Appendix, Fig. S2) (39, 40). The first group comprises UKH-1114, which alleviates mechanical hypersensitivity in a mouse model of neuropathic pain (3) and reduces neuronal toxicity in a model of Huntington’s disease (20). Based on the positive outcomes using UKH-1114 as a treatment in models of neuropathic pain, we modified its chemical structure to identify less lipophilic analogs with improved binding profiles and physicochemical properties. We synthesized FEM-1689 (SI Appendix, Fig. S3), which has one less methylene group than UKH-1114, and found it to be a highly selective compound with improved physicochemical properties (Fig. 2). FEM-1689 is >100-fold more selective for σ2R/TMEM97 than 40 CNS proteins except for σ1R (10-fold) and norepinephrine transporter (NET; 24-fold) (SI Appendix, Table S1).

Structure, binding profiles, physicochemical properties, and pharmacokinetic parameters for FEM-1689. Values are reported as averages ± SD.
Antinociceptive Effect of FEM-1689 in Male and Female Mice Requires an Intact Tmem97 Gene.
An important unresolved issue is whether σ2R/TMEM97 ligands reduce pain hypersensitivity specifically through action on σ2R/TMEM97. To address this question, we used a global TMEM97-KO mouse. Male and female TMEM97KO animals and their wild-type counterparts had similar paw mechanical sensitivity (von Frey), paw heat sensitivity (Hargreaves assay), and paw cold sensitivity (acetone) responses suggesting that the loss of TMEM97 did not alter their baseline sensation (SI Appendix, Fig. S4). We then examined whether TMEM97KO animals develop an enhanced or blunted mechanical neuropathic pain phenotype following SNI (41) (Fig. 3A). Male and female TMEM97KO and wild-type animals developed severe and prolonged mechanical hypersensitivity following SNI when tested at days 7, 10, and 14 after nerve injury (Fig. 3 B and C). When animals were tested 30 d following nerve injury, they were still mechanically hypersensitive. Male and female wild-type and TMEM97KO animals were then treated with a single intravenous injection of FEM-1689 dosed at 10 mg/kg, a dose determined based on previous studies with the structurally similar σ2R/TMEM97 ligand, UKH-1114 (3). We assessed TMEM97KO and wild-type evoked mechanical thresholds daily for 7 d and found little or no change for mechanical sensitivity in either group (Fig. 3 D and E). Animals were allowed to recover for 2 wk before a second treatment with a higher 20 mg/kg dose of FEM-1689. We found that this higher dose effectively reversed mechanical hypersensitivity in both male and female wild-type mice for roughly 4 d with a single administration. Notably, FEM-1689 failed to reduce mechanical hypersensitivity in TMEM97KO mice, showing that the effects of FEM-1689 are dependent on σ2R/TMEM97. The effect size measurements for FEM-1689 were quantified for males (Fig. 3F) and females (Fig. 3G).
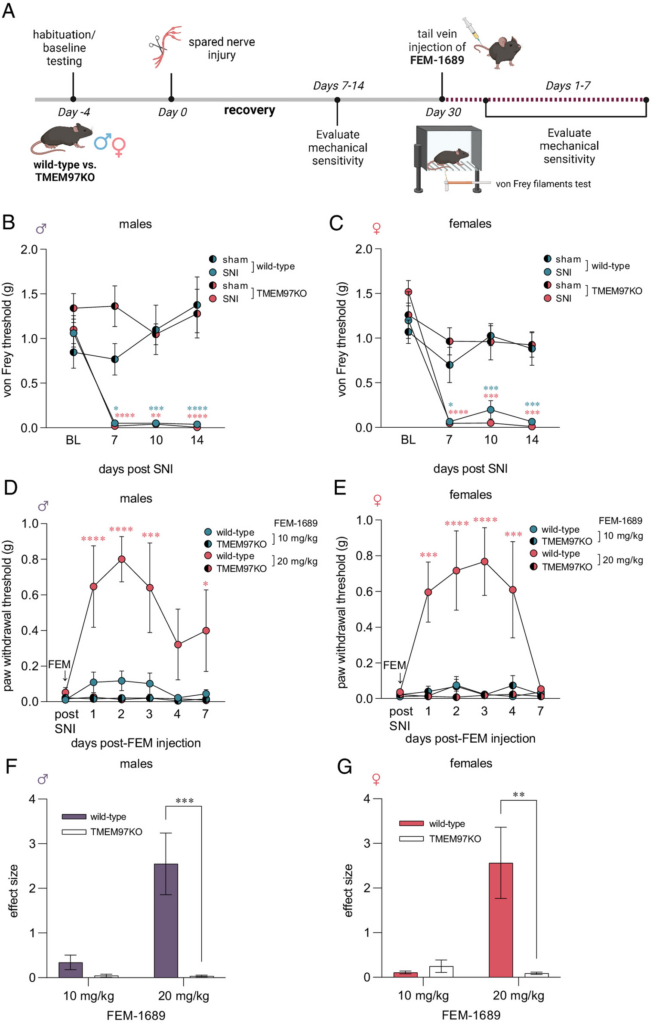
Mechanical pain hypersensitivity following SNI in wild-type and global TMEM97-KO mice. (A) Experimental paradigm. Mechanical hypersensitivity was assessed using the von Frey filaments test at baseline prior to SNI and for 14 d postsurgery. Mice were treated with FEM-1689 (10 mg/kg) intravenously 30 d after SNI surgery and assessed for mechanical hypersensitivity for the following 7 d. Another intravenous injection of FEM-1689 (20 mg/kg) was given 2 wk later and mechanical hypersensitivity assessed for 7 d. (B and C) Following SNI, no significant difference in mechanical hypersensitivity between wild-type and TMEM97KO littermates of both sexes was observed, suggesting that TMEM97 did not contribute to the development of neuropathic pain following nerve injury. Wild-type SNI (male n = 6, female n = 5), wild-type sham (male n = 6, female n = 5), TMEM97KO SNI (male n = 5, female n = 5), TMEM97KO sham (male n = 5, female n = 5). (D and E) A single 20 mg/kg intravenous injection of FEM-1689 reversed mechanical hypersensitivity in wild-type (male n = 6, female n = 5) but not TMEM97KO (male n = 5, female n = 5) mice. A lower dose of 10 mg/kg was not sufficient to reduce mechanical hypersensitivity in these animals. Repeated measures two-way ANOVA with Holm-Sidak’s multiple comparison test, *P < 0.05, **P < 0.01, ***P < 0.001, and ****P < 0.0001. (F and G) Effect size analysis demonstrates that a higher dose of 20 mg/kg of FEM-1689 provides maximal antinociceptive effect. Two-way ANOVA with Sidak’s multiple comparison test, **P < 0.01 and ***P < 0.001. Blue and red asterisks indicate wild-type and TMEM97KO groups compared to sham controls in (B and C) and wild-type vs. TMEM97KO groups in (D and E).
FEM-1689 Inhibits the ISR and Promotes Neurite Outgrowth in a σ2R/TMEM97-Dependent Fashion.
The next phase of our investigations was directed toward elucidating the mechanism by which FEM-1689 acts on sensory neurons by binding to σ2R/TMEM97. Prior work has suggested a link between σ2R/TMEM97 and cholesterol synthesis and trafficking, processes that are heavily regulated by 5′ adenosine monophosphate-activated protein kinase (AMPK) and its substrate, acetyl-CoA carboxylase (42). AMPK agonists are also known to produce antinociception in mouse models (22, 43–46). We treated cultured mouse DRG neurons with FEM-1689 at concentrations covering a range (10, 100, and 1,000 nM), which is consistent with target binding (Ki = 17±8 nM) over the course of 16 h, and found no change in p-ACC levels, whereas A769662 (100 µM), a known AMPK activator, increased p-ACC levels in both wild-type and TMEM97KO neurons (SI Appendix, Fig. S5 A and B). Our observation suggests that FEM-1689 does not influence the AMPK-p-ACC pathway and that a different antinociceptive pathway must be involved.
We then tested the hypothesis that FEM-1689 would inhibit the ISR in DRG neurons. Multiple lines of evidence support this hypothesis: 1) σ2R/TMEM97 and ISR transducers like protein kinase R-like ER kinase (PERK) are located on the ER membrane (40, 47); 2) a recent report on the effect of 20(S)-hydroxycholesterol on σ2R/TMEM97 implicated the ER-Golgi network (12); and 3) the ISR is engaged in trauma-induced and diabetic neuropathic pain conditions in DRG neurons (25, 26). Accordingly, we cultured mouse DRG neurons from wild-type and TMEM97KO animals, treated them with FEM-1689 over 16 h, and measured changes in the levels of p-eIF2α using immunocytochemistry (ICC) (Fig. 4 A and B). Measured with ICC, basal levels of p-eIF2α were much lower in TMEM97KO neurons than their wild-type counterparts. Treatment of wild-type neurons with ISRIB (200 nM), a well-known ISR inhibitor, reduced p-eIF2α levels to the same extent as p-eIF2α levels in vehicle- and ISRIB-treated TMEM97KO neurons (Fig. 4C). FEM-1689 reduced p-eIF2α levels, as compared to vehicle-treated cells, in wild-type mouse DRG neurons but not in DRG neurons cultured from TMEM97KO animals (Fig. 4 D and E). We found that FEM-1689 maximally inhibited the ISR at 100 nM to the same extent as ISRIB (200 nM) (Fig. 4D) (48). We calculated the p-eIF2α IC50 of FEM-1689 to be 30 nM in wild-type mouse DRG neurons, which was very similar to the binding affinity of FEM-1689 to TMEM97 (Ki = 17 ± 8 nM).
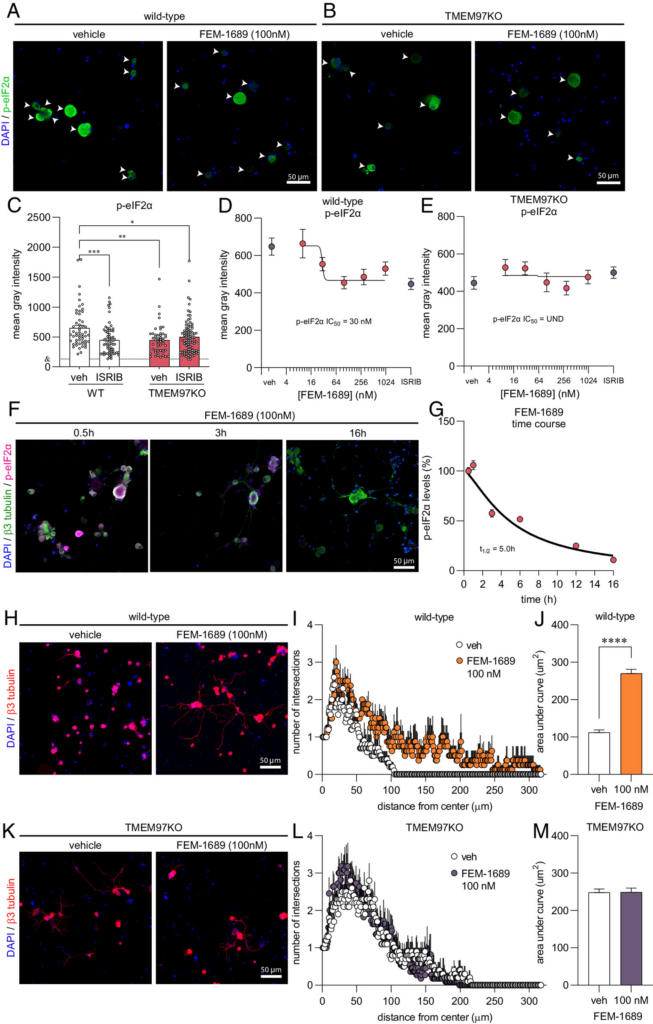
FEM-1689 reduces p-eIF2α levels and promotes neurite outgrowth in vitro. (A and B) Cultured mouse DRG neurons obtained from wild-type and TMEM97KO animals were treated with FEM-1689 over 16 h. (C) Basal levels of p-eIF2α were lower in TMEM97KO neurons. ISRIB (200 nM) treatment reduced p-eIF2α levels in wild-type neurons but failed to change p-eIF2α immunoreactivity in TMEM97KO neurons. (D and E) Immunoreactivity of p-eIF2α was assessed across five doses (10, 30, 100, 300 nM, and 1 µM), and a dose–response curve was generated. An IC50 of 30 nM was determined with maximal effect at 100 nM which was comparable to ISRIB (200 nM) treatment. TMEM97KO DRG neurons did not respond to FEM-1689 treatment. (F and G) Wild-type mouse DRG neurons were treated with FEM-1689 (100 nM) at 0.5, 1, 3, 6, 12, and 16 h. We calculated a half-life (t1/2) for FEM-1689 of 5.0 h at reducing p-eIF2α levels in vitro. Maximal effect of FEM-1689 was observed at 16 h of treatment. (H–M) Sholl analysis of mouse DRG neurons following 100 nM of FEM-1689 treatment showed an increase in the number and complexity of neurites in wild-type neurons but not in TMEM97KO neurons. Area under the curve of Sholl analysis was used to statistically demonstrate this effect. Immunoreactivity against β3-tubulin was used to identify neuronal cell bodies and neurites. Arrows indicate neuronal cell bodies. ***P < 0.001 and ****P < 0.0001, two-tailed Student’s t test. UND = undetermined and & = average mean gray intensity of the primary antibody omission control.
We further characterized the temporal dynamics of FEM-1689 in reducing p-eIF2α levels by treating wild-type mouse DRG neurons with 100 nM FEM-1689 over a time period of 0.5, 1, 3, 6, 12, and 16 h and quantifying immunoreactivity of p-eIF2α (Fig. 4F). Using this approach, we calculated a half-life of 5.0 h for FEM-1689 in reducing p-eIF2α levels in vitro. Maximum inhibition of ISR was observed 16 h after treatment of mouse DRG neurons with FEM-1689. This extended time period is consistent with the delayed and prolonged antinociceptive effects of FEM-1689. FEM-1689 did not reduce levels of BiP, a chaperone important for initiating ER stress and ISR, in either wild-type or TMEM97KO neurons (SI Appendix, Fig. S5 C and D). Sholl analysis of cultured mouse DRG neurons showed that FEM-1689 promoted neurite outgrowth in wild-type neurons but not in TMEM97KO neurons (Fig. 4 F–K). Notably, neurite outgrowth in vehicle-treated TMEM97KO neurons was more pronounced than vehicle-treated wild-type neurons—an observation that may be due to reduced ISR in TMEM97KO cells and hence, enhanced protein synthesis. These data show that σ2R/TMEM97 is necessary for FEM-1689 to reduce ISR and promote neurite outgrowth.
Norbenzomorphan σ2R/TMEM97 Ligands SAS-0132 and DKR-1677 Enhance the ISR.
The next question we addressed was whether other compounds that bind to σ2R/TMEM97 inhibit the ISR and whether ISR inhibition is specific to σ2R/TMEM97 modulators that promote antinociception. We have suggested that dissimilar biological outcomes of σ2R/TMEM97 modulators may arise from differences in the way individual ligands bind and interact with the protein binding pocket (40). The homologous piperazine-substituted norbenzomorphans SAS-0132 and DKR-1677 (SI Appendix, Fig. S2) represent a chemotype that is structurally distinct from the aryl-substituted norbenzomorphan FEM-1689. Computational docking studies of SAS-0132 and FEM-1689, which were performed using the published structure of σ2R/TMEM97 (4), predict that these two modulators interact differently with the extended binding site of σ2R/TMEM97 (SI Appendix, Fig. S6). SAS-0132, which is neuroprotective but has no antinociceptive effect on its own, was previously shown to inhibit the antinociceptive effects of UKH-1114, which is a homolog of FEM-1689 (3, 17). This observation is consistent with the hypothesis that individual σ2R/TMEM97 modulators may exert distinct, sometimes opposing, biological effects (3). Indeed, after treatment of wild-type mouse DRG neurons with 10, 30, 100, and 300 nM of SAS-0132 or DKR-1677 over 16 h, we found that both compounds promote the phosphorylation of eIF2α, in contrast to the inhibitory effects of FEM-1689 (Fig. 5). These data suggest that distinct chemotypes of σ2R/TMEM97 binding compounds have differing effects on the ISR, potentially mediated by how they interact with σ2R/TMEM97.
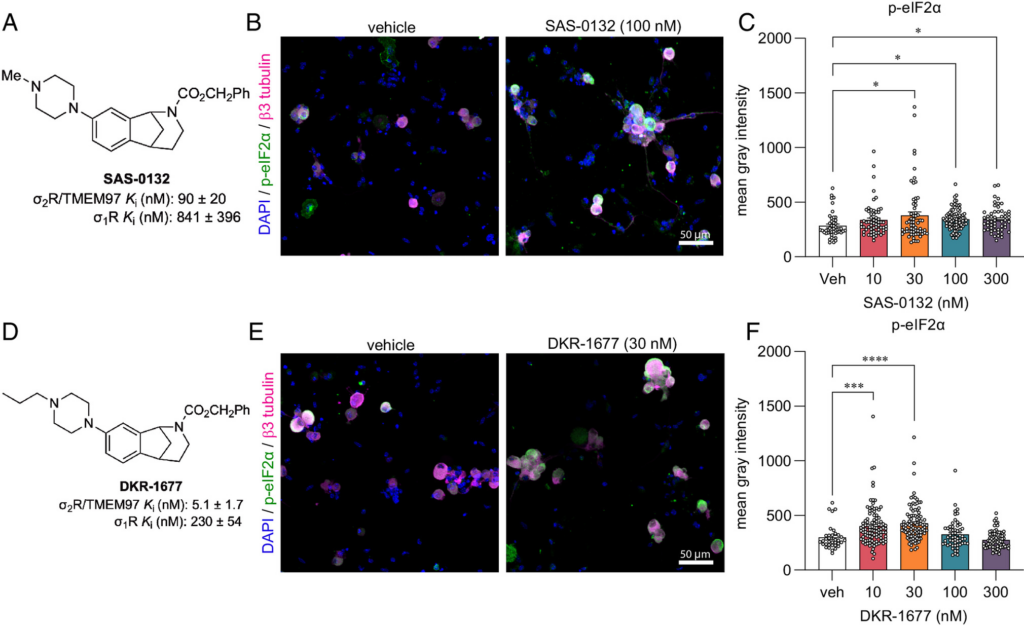
Norbenzomorphans such as SAS-0132 (A–C) and DKR-1677 (D–F) stimulate the ISR by promoting the phosphorylation of eIF2α in cultured wild-type mouse DRG neurons. Cells were treated for 16 h with either SAS-0132 or DKR-1677 at 10, 30, 100, or 300 nM concentrations. Data are presented as fold-change compared to the fluorescence measured in the vehicle-treated neurons. *P < 0.05, ***P < 0.001, and ****P < 0.0001, one-way ANOVA with Tukey’s post hoc test.
FEM-1689 Inhibits the ISR in HEK293T Cells.
Human embryonic kidney (HEK) 293 T cells express σ2R/TMEM97 [The Human Protein Atlas (49)], so we queried whether FEM-1689 would inhibit the ISR in this cell line. We found a concentration-dependent reduction in p-eIF2α immunoreactivity following FEM-1689 treatment for either 2 or 16 h using ICC and spectrophotometry (Fig. 6 A and B). We tested the effect of FEM-1689 over nine concentrations, ranging from 0.1 nM to 1,000 nM, and measured p-eIF2α IC50 of FEM-1689 in HEK cells to be 5.9 and 0.7 nM for 2- and 16-h treatment conditions, respectively (Fig. 6 A and B). We found that the 2-h FEM-1689 incubation period produced a curve with sigmoidal characteristics. We examined the effects of FEM-1689 treatment on a broader panel of ISR-related proteins using western blotting. HEK293T cells treated with FEM-1689 over 16 h showed a concentration-dependent reduction in phosphorylation of eIF2α, eIF2A, and p-PERK, while BiP expression remained stable (Fig. 6 C–K). Our data show that FEM-1689 influences the PERK-eIF2α arm of the ISR in HEK293T cells and that HEK293 T cells can be used to develop a drug screening platform for pain therapeutics that target σ2R/TMEM97.
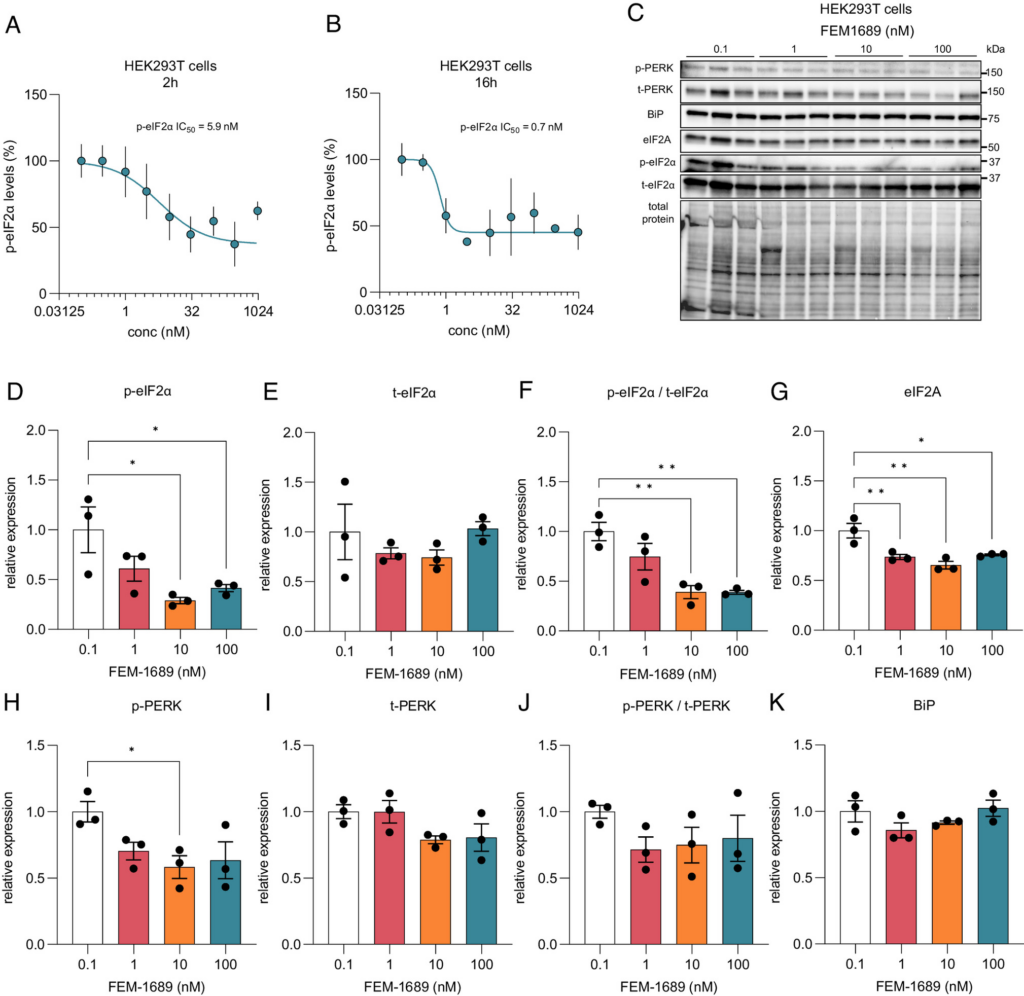
(A and B) HEK293T cells were treated for 2 and 16 h with FEM-1689 for across a range of nine concentrations (0.1, 0.3, 1, 3, 10, 30, 100, 300, and 1,000 nM) in 3 to 5 replicates. P-eIF2α levels were measured using ICC and spectrophotometry. IC50 was calculated to be 5.89 nM for the 2-h treatment and 0.74 nM for the 16-h treatment. FEM-1689 demonstrated time-dependent effect in reducing p-eIF2α levels in HEK293T cells. (C–K) In a separate experiment, HEK293T cells treated with FEM-1689 (0.1, 1, 10, and 100 nM) overnight were used for western blot analysis. Western blots showed a significant reduction in p-eIF2α, eIF2A, and p-PERK levels following FEM-1689 treatment suggesting the involvement of the PERK arm of ISR. BiP levels remained unchanged. One-way ANOVA with Tukey’s post hoc analysis, *P < 0.05 and **P < 0.01.
FEM-1689 Reverses Methylglyoxal-Induced Mechanical Hypersensitivity.
Methylglyoxal (MGO) is a metabolic by-product of glycolysis that is implicated in diabetic neuropathic pain and other painful neurodegenerative conditions (50–54). We have previously demonstrated that MGO induces an ISR response linked to mechanical hypersensitivity in rodents (26). We sought to determine whether the effect of FEM-1689 on reducing levels of p-eIF2α can alleviate ISR-dependent mechanical hypersensitivity caused by MGO. We treated wild-type mice with a single intraplantar injection of MGO (20 ng) to produce mechanical hypersensitivity lasting 6 d (Fig. 7A). A group of animals were treated with either FEM-1689 (20 mg/kg, IV) or ISRIB (2.5 mg/kg, intraperitoneal, IP) 24 h following MGO injection. FEM-1689 completely reversed MGO-induced mechanical hypersensitivity over the remaining time course of the experiment whereas the effect of ISRIB was transient (Fig. 7B). Our data show that FEM-1689 can completely reverse ISR-dependent pain hypersensitivity caused by MGO, demonstrating the utility for developing σ2R/TMEM97 modulators for diabetic neuropathic pain.
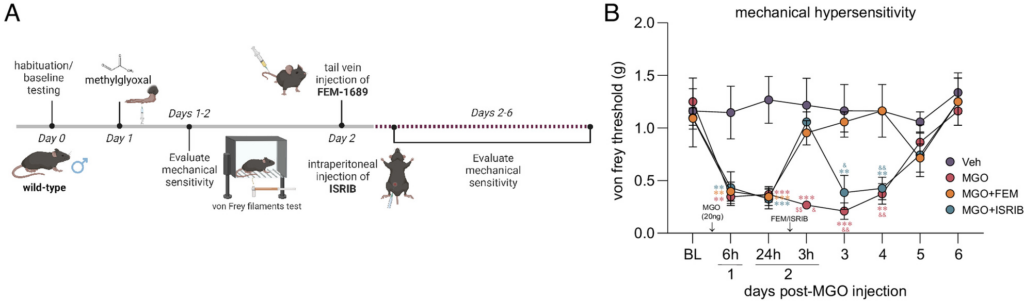
(A) MGO (20 ng) injection in the hind paw induces ISR-dependent mechanical pain hypersensitivity in wild-type mice over a course of 6 d. Mice were treated with FEM-1689 (20 mg/kg, IV) or ISRIB (2.5 mg/kg, IP) 24 h following MGO administration. (B) FEM-1689 and ISRIB reversed mechanical hypersensitivity in MGO-treated mice. ISRIB’s antiallodynic effects were observed 3 h after drug administration and reverted to a hypersensitive state 24 h after injection. FEM-1689 alleviated mechanical hypersensitivity for the duration of the pain state. *P < 0.05, **P < 0.01, and ***P < 0.001, repeated measures two-way ANOVA with Tukey’s post hoc test. Asterisk (*), dollar sign ($), and ampersand (&) denote post hoc comparison with vehicle (*), MGO+ISRIB ($), and MGO+FEM (&), respectively.
FEM-1689 Reduces p-eIF2α and Reverses MGO-Induced ISR Activity in Human Sensory Neurons.
To extend our findings with rodent DRG findings to humans, we treated cultured human DRG neurons from organ donors with FEM-1689 for 16 h. Consistent with our mouse data, we found that FEM-1689 significantly reduced p-eIF2α levels in human neurons at concentrations of 10 and 100 nM (Fig. 8 A and B). We then assessed whether FEM-1689 could reverse pathological ISR activation in human DRG neurons. To induce ISR in vitro, we treated human neurons with MGO at 1 µM, a concentration found in the plasma of diabetic neuropathic pain patients (50). MGO treatment increased p-eIF2α levels in human DRG neurons and cotreatment with FEM-1689 (100 nM) prevented this increase (Fig. 8 C and D). These findings support the conclusion that ISR activation associated with stimuli that cause neuropathic pain in humans can be blocked by σ2R/TMEM97 modulation.
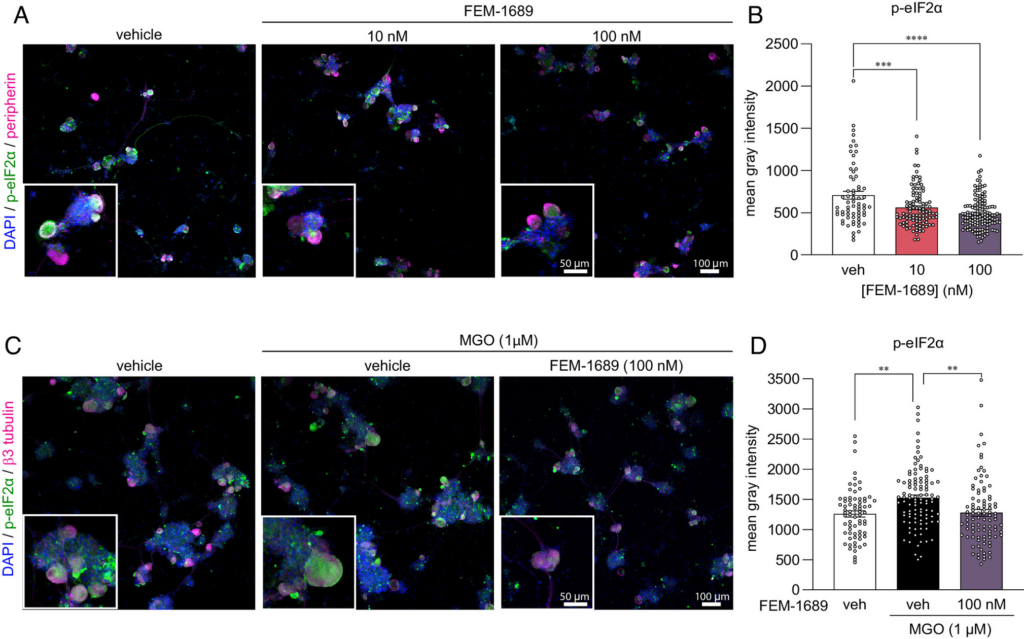
(A and B) FEM-1689 treatment (10 and 100 nM) of cultured human DRG neurons significantly reduced p-eIF2α levels. (C and D) MGO is known to induce the ISR. Cotreatment of human neurons with MGO (1 µM) and FEM-1689 (100 nM) prevented an increase in p-eIF2α suggesting that FEM-1689 limits the effect of MGO. Peripherin and β3-tubulin were used to identify DRG neurons. One-way ANOVA followed by Tukey’s post hoc test, **P < 0.01, ***P < 0.001, and ****P < 0.0001.
Discussion
Our experiments clearly demonstrate that the antinociceptive effects of a σ2R/TMEM97 ligand in mice of both sexes require direct modulation of σ2R/TMEM97, not σ1R or any other protein or receptor. This work also shows that modulation of σ2R/TMEM97 leads to the inhibition of the ISR in mouse and human DRG neurons. Because reduction of the ISR has been linked to pain relief (22, 26, 29), we posit that a plausible cellular mechanism for the antinociceptive effects of FEM-1689 and by extension σ2R/TMEM97 involves reducing the ISR. Finally, we show that human nociceptors express the TMEM97 gene, FEM-1689 reduces eIF2α phosphorylation in cultured human DRG neurons, and that the MGO-induced ISR in human neurons can be prevented using FEM-1689. These observations suggest that targeting σ2R/TMEM97 in pain patients could reduce mechanical hypersensitivity by inhibiting the ISR, a mechanism similarly observed in mice. We conclude that these findings nominate σ2R/TMEM97 as a bonafide target for developing effective treatments for neuropathic pain.
The time course of action of σ2R/TMEM97 modulators in mouse neuropathic pain models is different from many other antinociceptive compounds that have a rapid onset of action. Prior to the present study, the slow onset of these antinociceptive effects in the mouse SNI model was independently observed by two different groups using distinct classes of σ2R/TMEM97 ligands (3, 4). Herein, we have shown that this effect is mediated specifically by σ2R/TMEM97 because the antinociceptive activity is completely lost in male and female TMEM97KO mice. These observations suggest that the kinetics of signaling for σ2R/TMEM97 may be the primary underlying reason for the delayed response. The ISR is a major mechanism that controls long-lasting changes in gene expression by influencing the translation of key injury-induced transcription factors like activating transcription factor 4 (ATF4) and C/EBP homologous protein (CHOP). ISR-mediated changes involving multiple transcriptional and posttranslational modifications take time to initiate and progress. It is possible that this time course is responsible for the delay in the onset of action of FEM-1689 vs. the pharmacokinetics of ligand binding to σ2R/TMEM97. The molecular signaling pathways downstream of σ2R/TMEM97 modulation require further investigation.
There is an important factor that differentiates other studies of ISR inhibitors and our findings with FEM-1689. In previous studies (26, 29), we noted that ISR inhibitors ISRIB and 4-PBA alleviate mechanical hypersensitivity over a relatively shorter duration of efficacy (hours) in vivo than FEM-1689 (days). The discrepancy in the duration of efficacy of ISRIB and FEM-1689 may be due to differences in their mechanism of action upstream of the ISR pathway as well as the pharmacokinetics of each compound. ISRIB allosterically binds to and promotes the catalytic activity of eIF2B, a guanine nucleotide exchange factor necessary for the recycling of nonphosphorylated eIF2 complex (55–57). In conditions of prolonged and extensive ISR activation, the effects of ISRIB are blunted (58). It is unlikely that FEM-1689 shares the same ISR inhibiting mechanism as ISRIB. So, our observations suggest that FEM-1689 may provide a distinct, more long-term way of modulating ISR than what has been observed previously with ISRIB.
Over the course of our studies to formulate compounds that bind selectively to σ2R/TMEM97, we have found several chemotypes that exhibit beneficial effects in a number of animal models (40). One group comprises aryl-substituted methanobenzazocines such as UKH-1114 and JVW-1034 (SI Appendix, Fig. S2). UKH-1114 alleviates mechanical hypersensitivity following nerve injury (3), and it reduces neuronal toxicity induced by mutant huntingtin protein in a model of Huntington’s disease (20). The methanobenzazocine JVW-1034 not only reduces withdrawal behaviors in two rodent models of alcohol dependence (59, 60), but it also alleviates heightened pain sensitivity that is induced by chronic alcohol exposure in mice (60). Herein we report that FEM-1689, a close analog of UKH-1114, also alleviates mechanical hypersensitivity following nerve injury and in response to MGO treatment. Another structural class of σ2R/TMEM97 modulators include piperazine-substituted norbenzomorphans such as SAS-0132 and DKR-1677 (Fig. 2). For example, SAS-0132 is neuroprotective and improves cognitive performance in animal models of age-related neurodegeneration (17, 61). Notably, SAS-0132 also blocks the antinociceptive activity of UKH-1114 (3), suggesting different σ2R/TMEM97 binding compounds may have differing effects on nociception. DKR-1677, a homolog of SAS-0132, is protective in two different models of traumatic brain injury (TBI). It reduces axonal degeneration and provides dose-dependent enhancement of cognitive performance in the blast injury model of TBI, while it protects oligodendrocytes and cortical neurons in the controlled cortical impact model (19). DKR-1677 also protects retinal ganglion cells from ischemia/reperfusion injury (21). We demonstrate herein that SAS-0132 and DKR-1677 increase p-eIF2α expression in mouse DRG neurons, whereas FEM-1689 reduces p-eIF2α, suggesting that these compounds have distinct effects on the ISR. These observations provide the basis for developing a drug screening framework for effective pain therapeutics targeting σ2R/TMEM97.
Understanding how σ2R/TMEM97 modulators affect the ISR requires further investigation, but there are various clues for direct and indirect influence on the ISR in the literature. First, σ2R/TMEM97 localization to the ER membrane may link it to the ISR via ER stress, particularly by influencing eIF2α phosphorylation by the kinase PERK. Indeed, we observed a reduction in p-PERK in HEK cells following FEM-1689 treatment at concentrations where p-eIF2α and eIF2A are maximally reduced (Fig. 6), suggesting a possible link between σ2R/TMEM97 and the PERK pathway. Second, σ2R/TMEM97 is likely involved in transporting bioactive lipids, such as hydroxycholesterols (12), between cellular compartments, perhaps via a transporter activity like that of Niemann-Pick C1 (NPC1) protein (9). Excessive lipid intake and the demand for increase lipid synthesis promote lipid stress of the ER that is known to activate the PERK-eIF2α branch of the ISR and impair mitochondrial function (62–64). Finally, σ2R/TMEM97 regulates cellular Ca2+dynamics via its influence on store-operated calcium entry (11). The ER is the largest Ca2+ store in the cell and is sensitive to fluctuations in Ca2+ levels causing ER stress and ISR induction (65). It is currently unclear whether one or multiple mechanisms linked to inhibition of the ISR are required for antinociception associated with FEM-1689. However, discovery of this link to ISR inhibition enables further exploration of the downstream mechanistic actions of σ2R/TMEM97 modulators in DRG neurons.
Neurite outgrowth can be used to assess the neuromodulatory, neuroprotective, and neuroregenerative effects of drugs (66). Our findings demonstrate that FEM-1689 promotes neurite outgrowth and complexity in a σ2R/TMEM97-dependent manner as measured by Sholl analysis. Neurons lacking σ2R/TMEM97 also display enhanced neurite outgrowth compared to their wild-type counterparts without any drug treatment. This is explained by a reduced ISR level (Fig. 5C), and hence uninhibited protein synthesis in TMEM97KO neurons at basal levels. Axonal growth is a protein synthesis-demanding process so inhibition of the ISR in these conditions is consistent with an enhancement of protein synthesis driving this effect. Previous studies are also consistent with our findings as the ISR inhibitor ISRIB also enhances neurite outgrowth (67). Neuropathic pain models, like SNI, diabetic neuropathy, and chemotherapy-induced neuropathy are characterized by changes in axonal structure in the skin. A recent report demonstrates that aberrant reinnervation of mechanosensitive structures in the skin by nociceptors is a causative factor in late stages of neuropathic pain in mice (68). While we have not assessed skin innervation in the models we have tested, we have previously observed efficacy for σ2R/TMEM97 modulators in the SNI model at late time points where these skin innervation changes are observed (3). Given the effect we have seen on neurite outgrowth, it will be important to assess whether such effects can be caused in vivo with σ2R/TMEM97 ligands and whether they can lead to appropriate reinnervation of skin by nociceptors, avoiding aberrant targeting of Meissner’s corpuscles that is associated with neuropathic pain (68).
The findings reported herein provide insights regarding the mechanistic origin of the antinociceptive effects that can arise from modulating σ2R/TMEM97 with small molecules. There remain, however, unanswered questions. First, a definitive link between ISR activation and antinociception in vivo with FEM-1689 must be established. One way to do this would be to evaluate neuropathic pain in Eif2s1-KO mice. Such an experiment is complicated because loss of eIF2α, encoded by Eif2s1, or completely preventing the phosphorylation of eIF2α through a point mutation is lethal (69). Moreover, no specific antagonists of eIF2α have been described. The widely used classes of compounds that inhibit ISR are chemical chaperones, ISR kinase inhibitors, and eIF2B-stabilizers like ISRIB that do not directly target eIF2α and are not appropriate tools to answer this question. Second, it is unclear why TMEM97KO neurons have less p-eIF2α than WT neurons at baseline. This presents a paradox in which permanent “antagonism” of σ2R/TMEM97 (i.e., TMEM97KO) and σ2R/TMEM97 modulation by FEM-1689 both result in a reduction in p-eIF2α levels. This observation may suggest that FEM-1689 sequesters TMEM97 away from its binding partners and prevents the activation of the ISR, producing a KO-like effect. Additionally, the signaling effects of σ2R/TMEM97-binding compounds may depend on the pathway that is measured, for instance, LDL uptake was reduced to TMEM97KO levels in HeLa cell regardless of whether cells were treated with σ2R/TMEM97 “agonists” or “antagonists” (8). This was not the case in our observations on p-eIF2α levels. Third, it is important to acknowledge that FEM-1689 may have some polypharmacology as it appreciably binds to σ1 receptor (Ki = 167 ± 54 nM) and NET (Ki = 405 ± 227 nM), albeit with 10- and 24-fold less potency than σ2R/TMEM97 (Ki = 17 ± 8 nM). Since the antinociceptive effect of FEM-1689 in TMEM97KO mice is completely abrogated, we conclude that the effect of FEM-1689 in vivo is mediated by σ2R/TMEM97 and not σ1 receptor, NET, or other receptors. Moreover, σ1 receptor antagonists and NET inhibitors alleviate pain hypersensitivity over an acute (hours) or extensively prolonged (days to weeks) time period, respectively, neither of which fit our observations with FEM-1689 (33, 34, 65, 70–73). Nevertheless, at this stage, we cannot rule out the possibility of some polypharmacology with certainty. Fourth, it is important to determine whether the activity of FEM-1689 is due to a peripheral or central site of action. While our pharmacokinetic experiments show that the compound readily enters the brain (Fig. 2), we have also shown that FEM-1689 inhibits the ISR in the DRGs of mice and humans in vitro. Future experiments will use a nociceptor-specific TMEM97 KO mouse under development to address this important issue.